Effect of Gender on Development of Hippocampal Subregions From Childhood to Adulthood
- 1School of Psychology, Shenzhen University, Shenzhen, China
- 2Shenzhen Institute of Neuroscience, Shenzhen, China
The hippocampus is known to be comprised of several subfields, but the developmental trajectories of these subfields are under debate. In this study, we analyzed magnetic resonance imaging (MRI) data from a cross-sectional sample (198 healthy Chinese) using an automated segmentation tool to delineate the development of the hippocampal subregions from 6 to 26 years of age. We also examined whether gender and hemispheric differences influence the development of these subregions. For the whole hippocampus, the trajectory of development was observed to be an inverse-u. A significant increase in volume with age was found for most of the subregions, except for the L/R-parasubiculum, L/R-fimbria, and L-HATA. Gender-related differences were also found in the development of most subregions, especially for the hippocampal tail, CA1, molecular layer HP, GC-DG, CA3, and CA4, which showed a consistent increase in females and an early increase followed by a decrease in males. A comparison of the average volumes showed that the right whole hippocampus was significantly larger, along with the R-presubiculum, R-hippocampal-fissure, L/R-CA1, and L/R-molecular layer HP in males in comparison to females. Additionally, the average volume of the right hemisphere was shown to be significantly larger for the hippocampal tail, CA1, molecular layer HP, GC-DG, CA3, and CA4. However, for the presubiculum, parasubiculum, and fimbria, the left side was shown to be larger. In conclusion, the hippocampal subregions appear to develop in various ways from childhood to adulthood, with both gender and hemispheric differences affecting their development.
Introduction
The hippocampal cortex is an important structure in the limbic system, plays a critical role in memory, and is particularly vulnerable to the effects of aging (Richter-Levin, 2004; Malykhin et al., 2017; Zammit et al., 2017). The hippocampus formation is not a homogeneous structure and is comprised of several subfields with distinctive histological characteristics, including the subiculum (which can be further subdivided into the pre-subiculum, para-subiculum, and the subiculum proper), the four cornu ammonis sectors (CA1–4), and the dentate gyrus (Duvernoy, 2005). These subfields have been shown to play different roles in memory and learning (Yassa and Stark, 2011; Kesner, 2013; Reagh et al., 2014), and subsequently, are affected differently by Alzheimer’s disease (AD) and the normal process of aging (Thal et al., 2000; Mueller et al., 2007; Mak et al., 2017; Wang et al., 2018). Although age-related structural differences in hippocampal subfields have been previously examined (Yassa and Stark, 2011; Krogsrud et al., 2014), the segmentation was not fine-grained. Recently, a computational method for segmenting hippocampal subfields was presented (Iglesias et al., 2015), allowing for a finer differentiation. The atlas can be used to automatically segment the hippocampal subregions in structural magnetic resonance imaging (MRI) images, using an algorithm that can analyze multimodal data and adapt to variations in MRI contrast due to differences in acquisition hardware or pulse sequences. One study has already utilized this segmentation procedure, however, they did not distinguish between the left and right hemispheres (Tamnes et al., 2018). In this study, we utilized the more detailed segmentation method proposed by Iglesias et al. (2015) and examined the parasubiculum, presubiculum, subiculum, CA1, CA3, CA4, GC-DG, HATA, fimbria, molecular layer HP, fissure, and the tail in both the left and right hippocampus.
Despite the numerous lifespan studies that have focused on the developmental differences in the cerebral cortex (Sowell et al., 2003), little is known about the developmental trajectory of the hippocampal formation across its entire lifespan. Longitudinal investigations regarding hippocampal development have suggested that total hippocampal volume stabilizes in children (approximately at 4 years of age); a consistent and monotonic decline is evident in early adulthood, which shows a more rapid rate of decline in the seventh or eighth decades (Raz et al., 2010; Mattai et al., 2011; Sullivan et al., 2011). Results for the adolescent period have been more variable. Some longitudinal studies have found no significant age-related effects (Mattai et al., 2011; Sullivan et al., 2011). Numerous studies have observed volume increases (Dennison et al., 2013), decreases (Tamnes et al., 2013), or a quadratic inverted U-shaped trajectory (Wierenga et al., 2014; Narvacan et al., 2017; Herting et al., 2018). Thus, further examinations of the volume differences of the hippocampus at different ages in a cohesive sample from childhood to adulthood are needed.
Sexual dimorphism in brain development has been a focus of research for quite some time. With the explosion of neuroimaging research, adult male brains have been observed to be approximately 14% larger than female brains (Lenroot et al., 2007). However, sexual dimorphism in the hippocampus has generated a lot of controversies. Most reviews regarding gender-related differences in the human brain have stated that the hippocampus is larger in females than in males (Cahill, 2006; Hines, 2010). Some studies suggest that the hippocampus is larger in females, however, only during childhood (Cosgrove et al., 2007) or adolescence (Neufang et al., 2009). In contrast, other studies have noted greater hippocampal growth in males during adolescence (Suzuki et al., 2005; Bramen et al., 2011; Tamnes et al., 2018). Additionally, several studies support that the human hippocampus is not sexually-dimorphic (Tan et al., 2016). It is important to note that one factor that may be able to reconcile these published differences is age.
Hippocampal asymmetry and laterality have been observed in normal aging, with most reporting a right-greater-than-left asymmetry (Maller et al., 2007; Schmidt et al., 2018). From a molecular, structural, developmental, and sensitivity point of view, the left and the right hippocampus are completely unlike each other (Samara et al., 2011). However, there have been some discrepancies in the literature over the nature of this putative hippocampal asymmetry. Some studies suggest that the observed asymmetry is partly due to a visual perception bias if the volumes are manually traced (Maltbie et al., 2012; Rogers et al., 2012). Also, the subjects of this research mainly focus on adults and the elderly, but lack reports on adolescents. Therefore, in this study we analyzed a large cross-sectional sample comprised of 198 participants, 6–26 years of age, using a novel automated hippocampal segmentation tool. First, we characterize the developmental trajectory of the hippocampal subfields from childhood to adulthood. Second, we identify the differences in the developmental trajectories of the hippocampal subfields in the left and right hemispheres. Third, we investigate whether underlying gender-related differences are present in the volume of the hippocampal subfields from childhood to adulthood.
Materials and Methods
Subjects
This study included two datasets, comprised of a total of 198 healthy Chinese: (1) the first dataset included 89 Chinese participants aged 6–26 years (with an average age of 12.27 ± 6.46 years, 40 females, 49 males) that had been scanned at the Beijing MRI Center for Brain Research of the Chinese Academy; and (2) the second is a public dataset comprised of 109 Chinese participants (Typically-Developing Controls1) aged 8–15 years (with an average age of 11.15 ± 2.02 years, 46 females, 63 males) obtained from the Beijing site of the ADHD-200 dataset via the International Neuroimaging Data-sharing Initiative (Consortium, 2012). Of the total 198 participants, there were 86 females (with an average age of 11.57 ± 4.98) and 112 males (with an average age of 11.71 ± 4.31), the scatterplots of the ages are shown in Figure 1 and Supplementary Figure 1. For dataset 1, informed consent was obtained from the subjects and/or their parents as appropriate, and the study was approved by the Institutional Review Board of Beijing MRI Center for Brain Research. In dataset 2, the detailed information of the participants was accessed from the public data-sharing website of the ADHD-200 project1.
MRI Acquisition
For dataset 1, all of the MRIs were performed at the Beijing MRI Center for Brain Research of the Chinese Academy of Sciences using a 3 Tesla imager (Siemens, Erlangen, Germany) with a standard head coil. Three-dimensional, high-resolution anatomical scans were acquired using an MPRAGE sequence with the following parameters: TR = 2,300 ms, TE = 3.01 ms, TI = 1,000 ms, FA = 9°; 176 coronal T1-weighted slices with a acquisition matrix = 256 × 256, FOV = 256 × 240 mm2 and voxel sizes = 1 × 1 × 1 mm. In dataset 2, the images were acquired using a 3T Siemens Trio scanner with the following scanning parameters: T1-weighted magnetization-prepared rapid acquisition gradient-echo sequences, TR = 2,530 ms, TE = 3.39 ms, TI = 1,100 ms, FA = 7°, acquisition matrix = 256 × 256, FOV = 256 × 256 mm2, 128 slices, slice thickness = 1.33 mm, and average = 1.
Image Processing
Automated segmentation of the hippocampal subfields was performed using the hippo-subfields module in FreeSurfer 6.0, a well-validated open-source software suite that is freely available2. The technical details of this automated processing and the specific processing steps are described in detail elsewhere (Iglesias et al., 2015; Tamnes et al., 2018). The reported data were produced using the FreeSurfer default processing stream (recon-all), which includes transformation to Talairach space, intensity normalization for correction of magnetic field inhomogeneities, and removal of non-brain tissues (i.e., skull-stripping). We visually inspected the resulting surface models for motion artifact and manually edited the surfaces in cases where there was overinclusion of the skull, pial matter, or white matter. The hippocampus of each subject was segmented into 12 subfields for each hemisphere: parasubiculum, presubiculum, subiculum, CA1, CA3, CA4, GC-DG, HATA, fimbria, molecular layer HP, fissure, and the tail. The volume of each subfield and the ICV (Intra Cranial Vol) of each subject were then computed and extracted.
Harmonization Procedures
The removal of side effects from the different datasets was carried out using a posteriori harmonization statistical method named ComBat, which had initially been proposed for genomic studies to correct the so-called batch effect, and has been previously applied to image features from MRIs (Fortin et al., 2017, 2018). We used the “combat” R function provided3.
Statistical Analysis
Partial correlation analysis was used to test the influence of age and gender on each hippocampal subfield. First, we ran correlations between age and each subfield volume as well as the total hippocampal volume, controlling for ICV and gender. Then, we grouped each subfield volume into females and males, and ran correlations between age and volume for each group, controlling for ICV. Analysis of covariance (ANCOVA) was performed to analyze differences in the hippocampal volumes in both its entirety (whole) and its subfields between females and males, using age and ICV as covariates. Paired-samples t-test and Wilcoxon signed-rank test were used to analyze differences in the volumes of the hippocampal subregions between the left (L) and the right (R) hemispheres. All of the results were corrected using FDR correction in MATLAB. The significance level for all of the results was set at P < 0.05.
Results
Effect of Age on the Development of Hippocampal Subregions
The association between age and hippocampal volume is illustrated in Figures 2, 3. As shown in Figure 2, positive associations between age and volume are observed bilaterally for the whole hippocampus from 6 to 26 years of age (P < 0.001 for both left and right). For both of these volumes, the trajectory of their development was observed to be an inverse-u, in which the volume was shown to increase early, followed by slight decreases (Figure 2). Among these studies of the hippocampal subregions, our results showed significant corrected (P = 0.002 for L-CA3, P < 0.001 for the others) age-related volume increases for all of the subregions, except the L/R-parasubiculum, L/R-fimbria, and L-HATA (Figure 3). For the L/R-CA1, L/R-molecular layer HP, L/R-subiculum, L-presubiculum, and L/R-hippocampal-fissure, the developmental trajectories showed early increases, followed by sharp decreases. For the L-hippocampal tail, its development followed a consistent increase. For the R-hippocampal tail, R-presubiculum, L/R-GC-DG, L/R-CA3, L/R-CA4, and R-HATA, their development all showed early increases, followed by decelerating decreases.
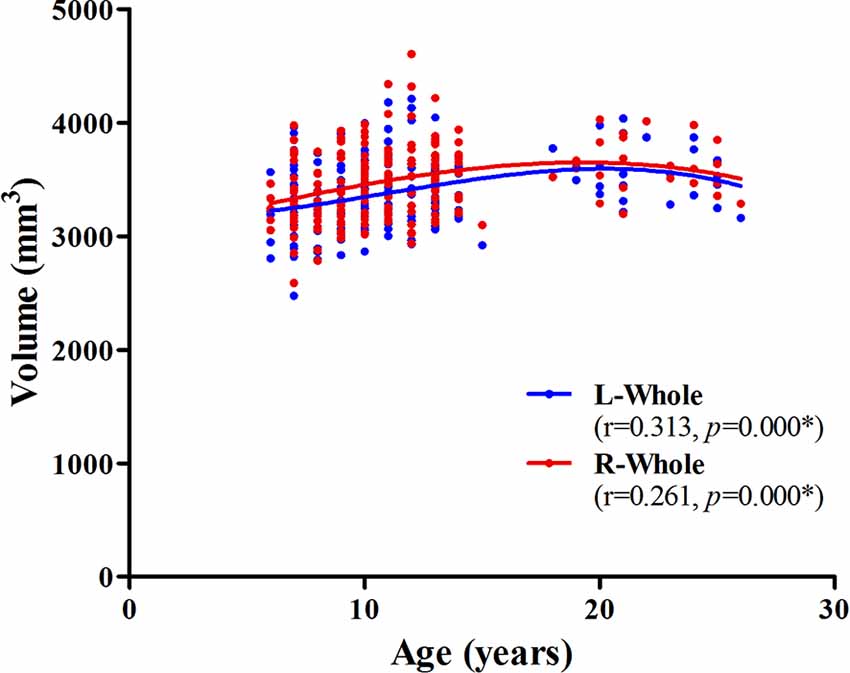
Figure 2. Scatterplots showing whole hippocampal volumes against age, using local smoothing models. Individual left (blue) and right (red) hippocampi are represented by individual lines. Volume is reported in mm3 and age is shown in years. L-Whole left whole hippocampus; R-Whole, the right whole hippocampus. *Indicates a significant relationship between volume and age after applying a 5% FDR correction.
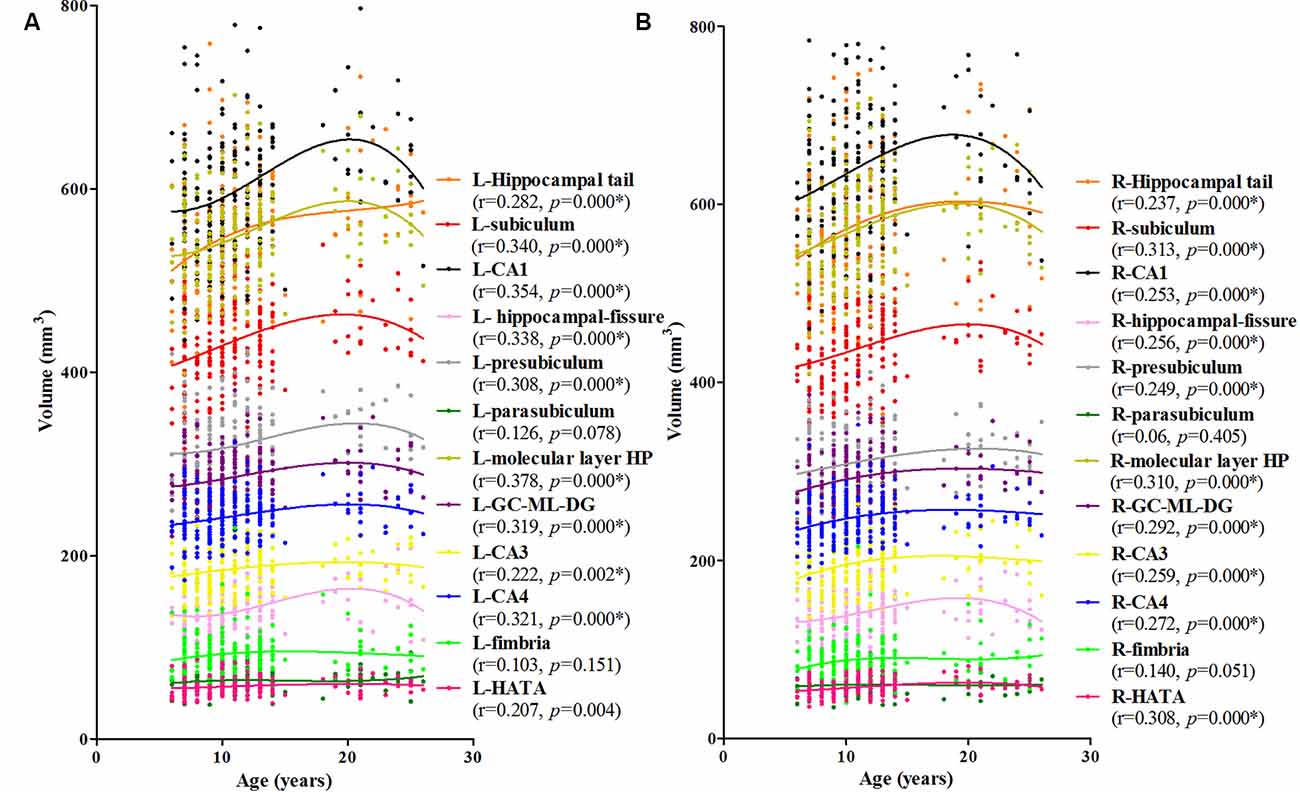
Figure 3. Scatterplots showing the volumes of hippocampal subfields against age, using local smoothing models. Panel (A) is the left hippocampus and panel (B) is the right hippocampus. Individual hippocampal subregions are represented by individual lines. Volume is reported in mm3 and age is shown in years. L-Whole left whole hippocampus; R-Whole, the right whole hippocampus. *Indicates a significant relationship between volume and age after applying a 5% FDR correction.
Effect of Gender on the Development of the Hippocampal Subregions
Left
For the females, a significant age-related volume increase was observed for the hippocampal tail, subiculum, CA1, hippocampal-fissure, presubiculum, molecular layer HP, GC-DG, CA3, and CA4 in the left hippocampus (9/12; Figure 4A). For the males, a significant age-related volume increase was found only in the subiculum, CA1, hippocampal-fissure, molecular layer HP, and GC-DG (5/12; Figure 4B). Interestingly, the developmental trajectories of the hippocampal tail, CA1, molecular layer HP, GC-DG, CA3, and CA4 were different between females and males; in females, there is a consistent increase whereas, in males, an early increase is observed followed by a decrease.
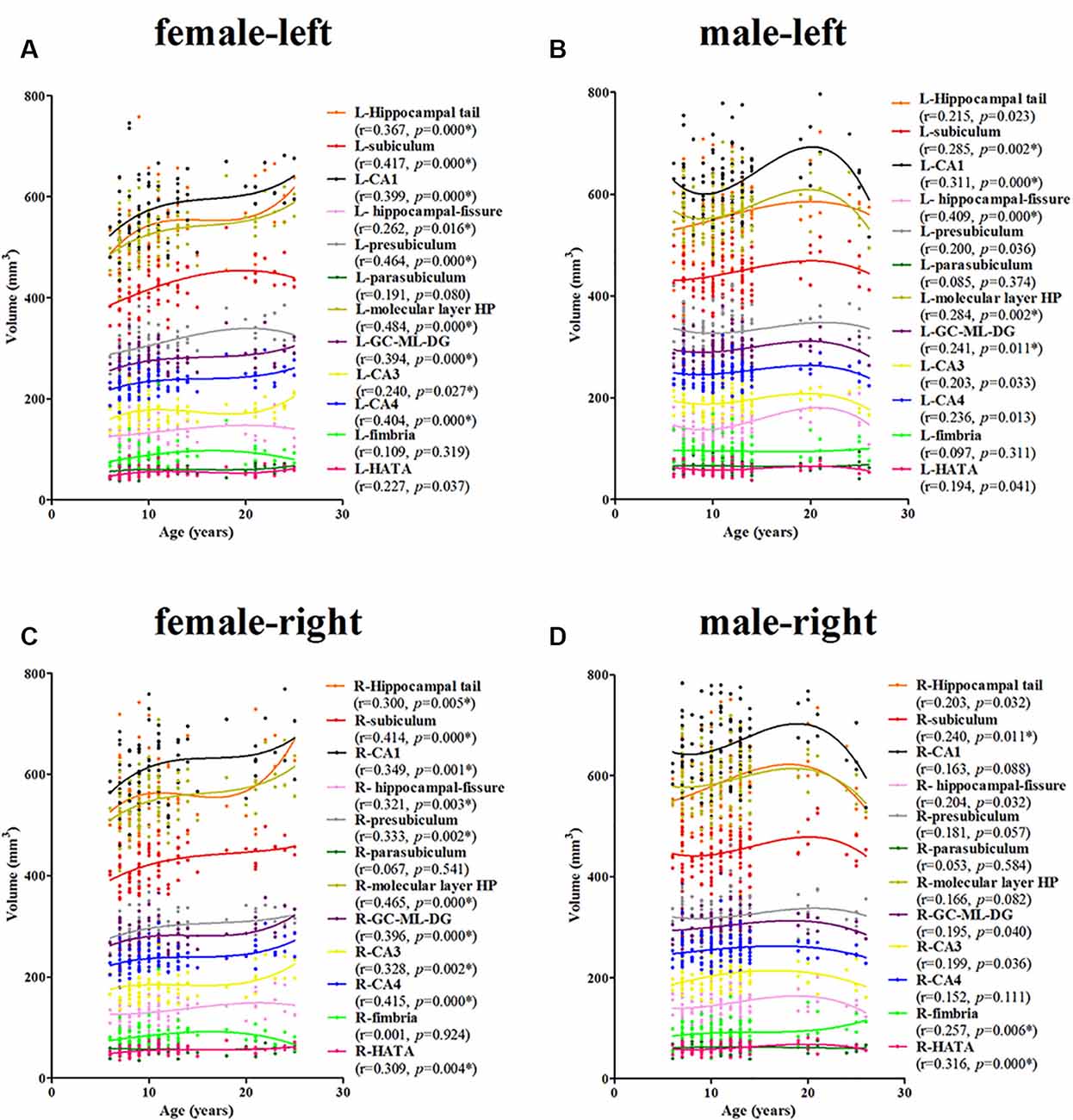
Figure 4. Scatterplots showing the volumes of the hippocampal subfields against age in females and males, using local smoothing models. Panel (A) is the left hippocampus in females, panel (B) is the left hippocampus in males, panel (C) is the right hippocampus in females and panel (D) is the right hippocampus in males. Individual hippocampal subregions are represented by individual lines. Volume is reported in mm3 and age is shown in years. L, left; R, right. *Indicates a significant relationship of volume with age after applying a 5% FDR correction.
Right
For the females, a significant age-related increase in the volume of the hippocampal tail, subiculum, CA1, hippocampal-fissure, presubiculum, molecular layer HP, GC-DG, CA3, CA4, and the HATA in the right hippocampus was observed (10/12; Figure 4C). For the males, a significant age-related volume increase was found only in the subiculum, fimbria, and the HATA (3/12; Figure 4D). The developmental trajectories were similar for the left subfields, except for the R-fimbria, which showed a consistent increase in males.
Effect of Hemisphere and Gender on Average Hippocampal Subregions Volume
Hemisphere
The averaged volumes of hippocampal subregions were compared between the left and right hemispheres. For the hippocampal tail, CA1, molecular layer HP, GC-DG, CA3, and CA4, the paired-samples T-Test indicated a significant difference between right and left hippocampal volume (P < 0.001), with the right hippocampal volume being larger than the left. Inversely, for presubiculum, parasubiculum, and fimbria, the left hippocampal volume was larger than the right, which showed a significant difference (P < 0.001). No difference was found for subiculum, hippocampal-fissure and HATA compared between left and right hemispheric volumes (Figure 5).
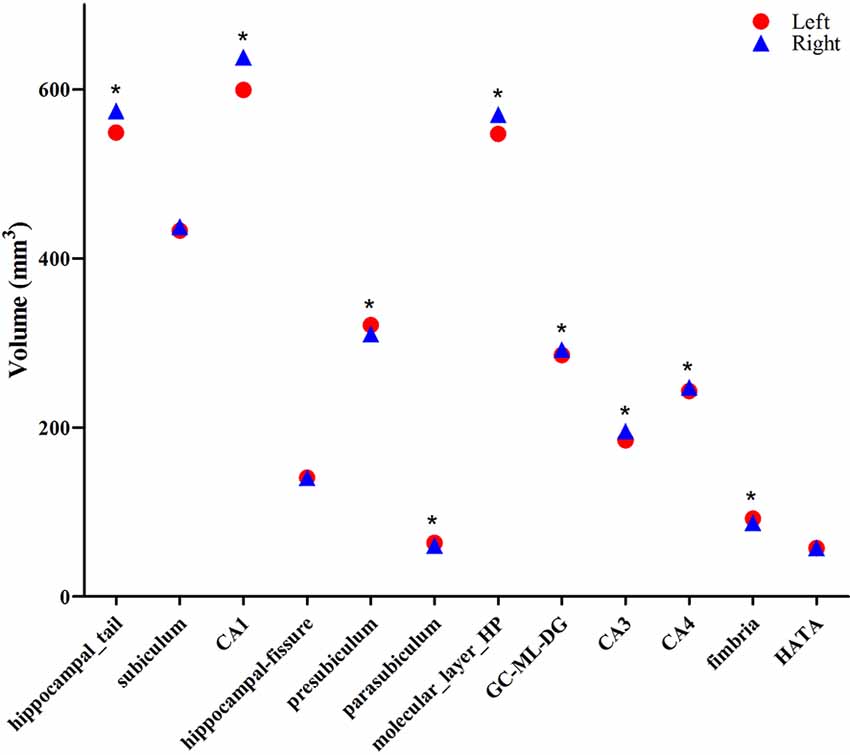
Figure 5. Comparison of the volumes of hippocampal subregions between the left (red circle) and right (blue triangle) hemispheres. *Indicates significant differences between left and right subfields after applying a 5% FDR correction.
Gender
The averaged hippocampal volumes were compared between females and males. The right hippocampus was significantly greater in males in comparison to females (P = 0.048; Figure 6). For averaged volumes of hippocampal subregions, a significantly greater volume was observed for the R-presubiculum (P = 0.016), L/R-CA1 (P = 0.024/P = 0.033), L/R-molecular layer HP (P = 0.035/P = 0.042), and the R-hippocampal-fissure (P = 0.044) in males in comparison to females (Figure 7). However, these differences were not significant following a 5% FDR correction.
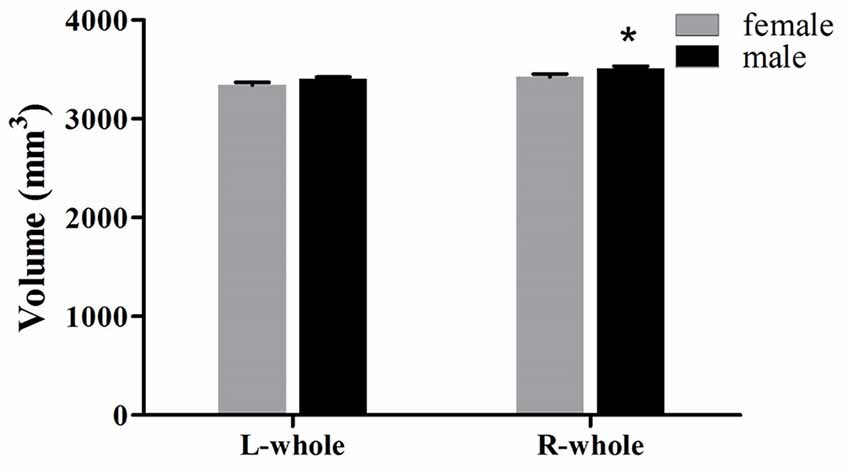
Figure 6. Comparison of whole hippocampal volumes (left and right) between females (gray) and males (black). L, left; R, right. *Indicates significant differences between females and males uncorrected (P < 0.05).
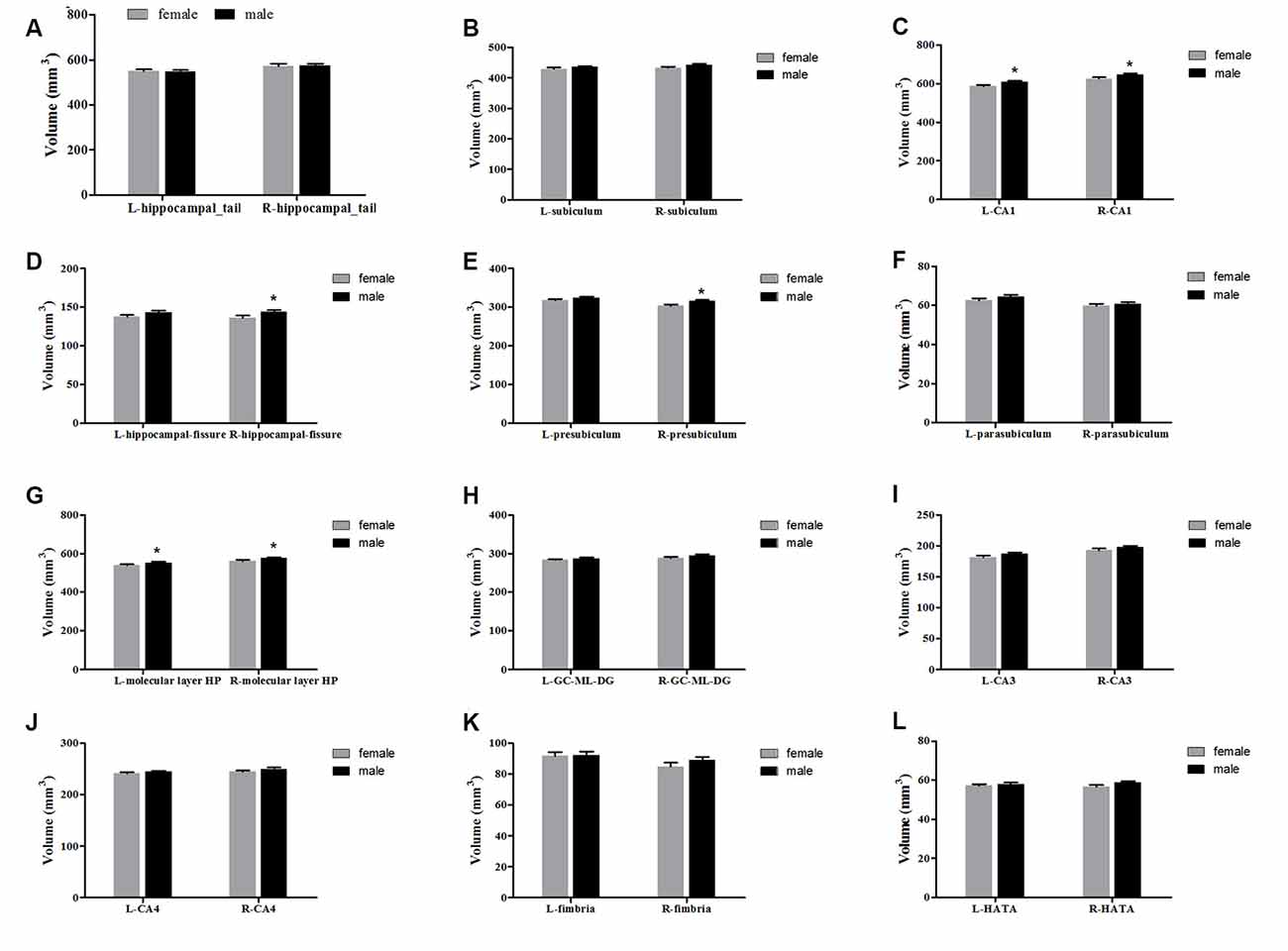
Figure 7. Comparison of the volumes of hippocampal subregions (left and right) between females (gray) and males (black). Panels (A–L) showed hippocampal_tail, subiculum, CA1, hippocampal-fissure, presubiculum, parasubiculum, molecular layer HP, GC-ML-DG, CA3, CA4, fimbria and HATA, respectively. L, left; R, right. *Indicates significant differences between females and males uncorrected (P < 0.05).
Discussion
Three important findings can be drawn from the results of this study: (1) For the whole hippocampus, the development of both the left and right hemispheres followed an inverted U-shaped trajectory from 6 to 26 years of age, however, the hippocampal subregions showed heterogeneous developmental patterns, with significant age-related volume increases for all subregions except for the L/R-parasubiculum, L/R-fimbria, and L-HATA; (2) the development of the hippocampal subregions showed sexual dimorphism from 6 to 26 years of age, showing a consistent increase in females and an early increase followed by a decrease in males for most subregions; and (3) the average volume of the hippocampal subregions between 6–26 years of age showed both gender and hemisphere related differences.
Many studies have investigated the developmental trajectory of the hippocampus from childhood to adolescence. In our present study, the whole hippocampal volume was shown to increase in late childhood and early adolescence, followed by a slight decrease in late adolescence and young adulthood, in agreement with the accumulating evidence from other studies (Wierenga et al., 2014; Coupe et al., 2017; Narvacan et al., 2017; Herting et al., 2018; Tamnes et al., 2018). However, quite a few reported studies on the development of the hippocampal subfields reported different trajectories (Krogsrud et al., 2014; Voineskos et al., 2015; Daugherty et al., 2016; Tamnes et al., 2018). Our results show significant age-related volume increases for the L/R-presubiculum, L/R-subiculum, L/R-CA1, L/R-CA3, L/R-CA4, L/R-GC-DG, R-HATA, L/R-molecular layer HP, L/R-fissure, and L/R-tail, which is in partial agreement with the previously reported studies. Krogsrud et al. (2014) reported that their models for the development of CA1, CA2/3, CA4/DG, presubiculum, and the subiculum estimated a gradually decelerating volume increase until 13–15 years of age, followed by little age-related changes; Lee et al. (2014) also showed that significant age-related increases in the subfield volumes were observed into early adolescence for the right CA3/DG and CA1. However, for the subiculum and fimbria, our results appear to be different from these two studies (Krogsrud et al., 2014; Lee et al., 2014). Also, Tamnes et al. (2018) used the same segmentation method that we used in this study, showing that nonlinear developmental trajectories, with early volume increases, were observed for the subiculum, CA1, and molecular layer; in contrast, the parasubiculum, presubiculum, CA2/3, CA4, and GC-DG showed linear volume decreases from 8 to 26 years of age. Unfortunately, they did not distinguish between the left and right hippocampal subregions. Thus, the differences between our results may be largely due to the variations in the hippocampal segmentation methods. Additionally, this raises the question of whether the developmental differences in the hippocampal subregions are constrained by differing developmental trajectories for the left vs. the right hippocampus.
Although the developmental trajectories for the left and right hippocampus are similar for all of the subregions, except for HATA, the average volume (between 6 and 26 years of age) for most of the hippocampal subregions are different. For 6/12 subregions (hippocampal tail, CA1, molecular layer HP, GC-DG, CA3, and CA4), the right hippocampal volume is larger than the left; for the other 3/12 (presubiculum, parasubiculum, and fimbria), a reverse pattern was identified. Although there are no strong reasons to predict hemispheric differences in hippocampal development, our results are consistent with previous studies, indicating more robust developmental differences in the right compared to the left hippocampus (Gogtay et al., 2006; DeMaster et al., 2014; Lee et al., 2014). DeMaster et al. (2014) found that adults had a smaller right hippocampal head, larger hippocampal body bilaterally, and smaller right hippocampal tail in comparison to children. Gogtay et al. (2006) reported more delayed developmental changes in the right, as compared to the left, hippocampus. Similarly, the right hippocampus was not larger than the left at the baseline, but increased more than the left during this developmental period, resulting in it being significantly larger at the later time-point (Dennison et al., 2013). Furthermore, asymmetries in the nature and extent of associations have been previously reported, with the right hippocampus being more likely to exhibit significant associations with a host of factors, including gender differences during puberty (Bramen et al., 2011). This study is the first to detect hemispheric differences in 12 hippocampal subregions between childhood and adulthood.
When the samples were separated by gender, the developmental trajectory for each subfield suggests some qualitative differences compared to the entire sample. Especially for the hippocampal tail, CA1, molecular layer HP, GC-DG, CA3, and CA4, in which a consistent increase is observed for females and an early increase followed by a decrease in males. Gender-related differences in the trajectory of hippocampal development is still a hotly debated topic. When estimating volume changes based on both age and pubertal development, the hippocampus increases in volume over puberty (7–20 years of age) in both females and males (Goddings et al., 2014). Similarly, several longitudinal studies have not found gender-related differences in developmental trajectories (Dennison et al., 2013; Wierenga et al., 2014; Tamnes et al., 2018). However, another study published by Neufang et al. (2009), which examined correlations between testosterone and brain volumes in boys and girls (8–15 years of age), reported that postpubertal males have significantly reduced hippocampal volumes compared to postpubertal females. Our present results are also consistent with this study, showing a decrease in volume after puberty in males in most hippocampal subregions. In addition to the above two views, a Meta-analysis revealed that the human hippocampus is not sexually-dimorphic. Meta-regression revealed no effect of age on the gender differences in the left, right, or bilateral hippocampus volumes (HCV; Tan et al., 2016). However, they did find that human males of all ages exhibit a larger HCV than females, but adjusting for individual differences in TBV or ICV results in no reliable gender-related differences. In the present study, we also adjusted the ICV in the statistical models, and the gender differences in the averaged hippocampal volumes are found for the right whole hippocampus, R-presubiculum, R-hippocampal-fissure, L/R-CA1, and L/R-molecular layer HP, which are greater in males as compared to females. Using the same segmentation method, Tamnes et al. (2018) also reported that boys had larger volumes than girls for all hippocampal subregions, except for the hippocampal fissure. Unlike us, they did not distinguish between the left and right hippocampus. Because of the hemispheric effect on hippocampal development, it is necessary to compare them separately. Regrettably, there is a gap in our data between 15–20 years old and the young adults might be under-represented. The “inverse-u” relationship observed in males but not in females might be affected by the uneven representations of the data points.
In conclusion, our results indicate that hippocampal subfields develop in various ways across childhood, adolescence, and early adult (6–26 years of age) with nonlinear trajectories, except for the L/R-parasubiculum, L/R-fimbria, and L-HATA. Additionally, gender differences in the development of most subregions were observed, especially for the hippocampal tail, CA1, molecular layer HP, GC-DG, CA3, and CA4, with a consistent increase in females and an early increase followed by a decrease in males. The averaged hippocampal volumes in the right whole hippocampus, R-presubiculum, R-hippocampal-fissure, L/R-CA1, and L/R-molecular layer HP are observed to be larger in males as compared to females. Finally, we also found that hemisphere differences exist in most hippocampal subregions, except for the subiculum, hippocampal-fissure, and HATA. In summary, our study is the first to examine the effects of age, sex, and hemisphere differences in the 24 hippocampal subfields from childhood to adulthood. Further studies are needed to confirm our findings and determine the value of these measurements for the differentiation between normal aging vs. other diseases, which affect the hippocampal subfields.
Data Availability Statement
The raw data supporting the conclusions of this article will be made available by the authors, without undue reservation.
Ethics Statement
The studies involving human participants were reviewed and approved by Institutional Review Board of Beijing MRI Center for Brain Research. Written informed consent to participate in this study was provided by the participants, and/or where necessary, the participants’ legal guardian/next of kin.
Author Contributions
LT and SM designed this study and revised and guided the experiment. SM wrote this manuscript and participated in the whole experiment process. BY and SM managed the whole experiment and analyzed the data. All authors contributed to the article and approved the submitted version.
Funding
This study was supported by the Shenzhen Double Chain Grant 256, the grants from Shenzhen basic research scheme (JCYJ20170818110103216, JCYJ20170412164413575, 2019SHIBS0003, JCYJ20170412111316339 and JCYJ20170412164259361); Guangdong key basic research program (2018B030332001); Guangdong Pearl River Talents Plan (2016ZT06S220); Shenzhen-Hong Kong Institute of Brain Science-Shenzhen Fundamental Research Institutions; National Science Foundation of China (81301063); China’s National Strategic Basic Research Program (973 program, 2012CB720701); and Shenzhen Peacock Plan (KQTD2015033016104926).
Conflict of Interest
The authors declare that the research was conducted in the absence of any commercial or financial relationships that could be construed as a potential conflict of interest.
Footnotes
- ^ http://fcon_1000.projects.nitrc.org/indi/adhd200/
- ^ http://surfer.nmr.mgh.harvard.edu/
- ^ https://github.com/Jfortin1/ComBatHarmonization
Supplementary Material
The Supplementary Material for this article can be found online at: https://www.frontiersin.org/articles/10.3389/fnhum.2020.611057/full#supplementary-material.
References
Bramen, J. E., Hranilovich, J. A., Dahl, R. E., Forbes, E. E., Chen, J., Toga, A. W., et al. (2011). Puberty influences medial temporal lobe and cortical gray matter maturation differently in boysthan girls matched for sexual maturity. Cereb. Cortex 21, 636–646. doi: 10.1093/cercor/bhq137
Cahill, L. (2006). Why sex matters for neuroscience. Nat. Rev. Neurosci. 7, 477–484. doi: 10.1038/nrn1909
Consortium, H. D. (2012). The ADHD-200 consortium: a model to advance the translational potential of neuroimaging in clinical neuroscience. Front. Syst. Neurosci. 6:62. doi: 10.3389/fnsys.2012.00062
Cosgrove, K. P., Mazure, C. M., and Staley, J. K. (2007). Evolving knowledge of sex differences in brain structure, function and chemistry. Biol. Psychiatry 62, 847–855. doi: 10.1016/j.biopsych.2007.03.001
Coupe, P., Catheline, G., Lanuza, E., Manjon, J. V., and Alzheimer’s Disease Neuroimaging Initiative (2017). Towards a unified analysis of brain maturation and aging across the entire lifespan: a MRI analysis. Hum. Brain Mapp. 38, 5501–5518. doi: 10.1002/hbm.23743
Daugherty, A. M., Bender, A. R., Raz, N., and Ofen, N. (2016). Age differences in hippocampal subfield volumes from childhood to late adulthood. Hippocampus 26, 220–228. doi: 10.1002/hipo.22517
DeMaster, D., Pathman, T., Lee, J. K., and Ghetti, S. (2014). Structural development of the hippocampus and episodic memory: developmental differences along the anterior/posterior axis. Cereb. Cortex 24, 3036–3045. doi: 10.1093/cercor/bht160
Dennison, M., Whittle, S., Yücel, M., Vijayakumar, N., Kline, A., Simmons, J., et al. (2013). Mapping subcortical brain maturation during adolescence: evidence of hemisphere- and sex-specific longitudinal changes. Dev. Sci. 16, 772–791. doi: 10.1111/desc.12057
Duvernoy, H. M. (2005). The Human Hippocampus: Functional Anatomy, Vascularization and Serial Sections With MRI. Berlin: Springer.
Fortin, J. P., Cullen, N., Sheline, Y. I., Taylor, W. D., Aselcioglu, I., Cook, P. A., et al. (2018). Harmonization of cortical thickness measurements across scanners and sites. NeuroImage 167, 104–120. doi: 10.1016/j.neuroimage.2017.11.024
Fortin, J. P., Parker, D., Tunc, B., Watanabe, T., Elliott, M. A., Ruparel, K., et al. (2017). Harmonization of multi-site diffusion tensor imaging data. NeuroImage 161, 149–170. doi: 10.1016/j.neuroimage.2017.08.047
Goddings, A. L., Mills, K. L., Clasen, L. S., Giedd, J. N., Viner, R. M., and Blakemore, S. J. (2014). The influence of puberty on subcortical brain development. NeuroImage 88, 242–251. doi: 10.1016/j.neuroimage.2013.09.073
Gogtay, N., Nugent, T. F., Herman, D. H., Ordonez, A., Greenstein, D., Hayashi, K. M., et al. (2006). Dynamic mapping of normal human hippocampal development. Hippocampus 16, 664–672. doi: 10.1002/hipo.20193
Herting, M. M., Johnson, C., Mills, K. L., Vijayakumar, N., Dennison, M., Liu, C., et al. (2018). Development of subcortical volumes across adolescence in males and females: a multisample study of longitudinal changes. NeuroImage 172, 194–205. doi: 10.1016/j.neuroimage.2018.01.020
Hines, M. (2010). Sex-related variation in human behavior and the brain. Trends Cogn. Sci. 14, 448–456. doi: 10.1016/j.tics.2010.07.005
Iglesias, J. E., Augustinack, J. C., Nguyen, K., Player, C. M., Player, A., Wright, M., et al. (2015). A computational atlas of the hippocampal formation using ex vivo, ultra-high resolution MRI: application to adaptive segmentation of in vivo MRI. NeuroImage 115, 117–137. doi: 10.1016/j.neuroimage.2015.04.042
Kesner, R. (2013). An analysis of the dentate gyrus function. Behav. Brain Res. 254, 1–7. doi: 10.1016/j.bbr.2013.01.012
Krogsrud, S. K., Tamnes, C. K., Fjell, A. M., Amlien, I., Grydeland, H., Sulutvedt, U., et al. (2014). Development of hippocampal subfield volumes from 4 to 22 years. Hum. Brain Mapp. 35, 5646–5657. doi: 10.1002/hbm.22576
Lee, J. K., Ekstrom, A. D., and Ghetti, S. (2014). Volume of hippocampal subfields and episodic memory in childhood and adolescence. NeuroImage 94, 162–171. doi: 10.1016/j.neuroimage.2014.03.019
Lenroot, R. K., Gogtay, N., Greenstein, D. K., Wells, E. M., Wallace, G. L., Clasen, L. S., et al. (2007). Sexual dimorphism of brain developmental trajectories during childhood and adolescence. NeuroImage 36, 1065–1073. doi: 10.1016/j.neuroimage.2007.03.053
Mak, E., Gabel, S., Su, L., Williams, G. B., Arnold, R., Passamonti, L., et al. (2017). Multi-modal MRI investigation of volumetric and microstructural changes in the hippocampus and its subfields in mild cognitive impairment, Alzheimer’s disease and dementia with Lewy bodies. Int. Psychogeriatr. 29, 545–555. doi: 10.1017/S1041610216002143
Maller, J. J., Anstey, K. J., Re’glade-Meslin, C., Christensen, H., Wen, W., and Sachdev, P. (2007). Hippocampus and amygdala volumesin a random community-based sample of 60-64 year olds and their relationship to cognition. Psychiatry Res. 156, 185–197. doi: 10.1016/j.pscychresns.2007.06.005
Maltbie, E., Bhatt, K., Paniagua, B., Smith, R. G., Graves, M. M., Mosconi, M. W., et al. (2012). Asymmetric bias in user guided segmentations of brain structures. NeuroImage 59, 1315–1323. doi: 10.1016/j.neuroimage.2011.08.025
Malykhin, N. V., Huang, Y., Hrybouski, S., and Olsen, F. (2017). Differential vulnerability of hippocampal subfields and anteroposterior hippocampal subregions in healthy cognitive aging. Neurobiol. Aging 59, 121–134. doi: 10.1016/j.neurobiolaging.2017.08.001
Mattai, A., Hosanaagar, A., Weisinger, B., Greenstein, D., Stidd, R., Clasen, L., et al. (2011). Hippocampal volume development in healthy siblings of childhod-onset schizophrenia patients. Am. J. Psychiatry 168, 427–435. doi: 10.1176/appi.ajp.2010.10050681
Mueller, S. G., Stables, L., Du, A. T., Schuff, N., Truran, D., Cashdollar, N., et al. (2007). Measurement of hippocampal subfields and age-related changes with high resolution MRI at 4T. Neurobiol. Aging 28, 719–726. doi: 10.1016/j.neurobiolaging.2006.03.007
Narvacan, K., Treit, S., Camicioli, R., Martin, W., and Beaulieu, C. (2017). Evolution of deep gray matter volume across the human lifespan. Hum. Brain Mapp. 38, 3771–3790. doi: 10.1002/hbm.23604
Neufang, S., Specht, K., Hausmann, M., Gunturkun, O., Herpertz-Dahlmann, B., Fink, G. R., et al. (2009). Sex differences and the impact of steroid hormones on the developing human brain. Cereb. Cortex 19, 464–473. doi: 10.1093/cercor/bhn100
Raz, N., Ghisletta, P., Rodrigue, K. M., Kennedy, K. M., and Lindenberger, U. (2010). Trajectories of brain aging in middle-aged and older adults: regional and individual differences. NeuroImage 51, 501–511. doi: 10.1016/j.neuroimage.2010.03.020
Reagh, Z. M., Watabe, J., Ly, M., Murray, E., and Yassa, M. A. (2014). Dissociated signals in human dentate gyrus and CA3 predict different facets of recognition memory. J. Neurosci. 34, 13301–13313. doi: 10.1523/JNEUROSCI.2779-14.2014
Richter-Levin, G. (2004). The amygdala, the hippocampus and emotional modulation of memory. Neuroscientist 10, 31–39. doi: 10.1177/1073858403259955
Rogers, B. P., Sheffield, J. M., Luksik, A. S., and Heckers, S. (2012). Systematic error in hippocampal volume asymmetrymeasurementisminimalwith amanual segmentation protocol. Front. Neurosci. 6:179. doi: 10.3389/fnins.2012.00179
Samara, A., Vougas, K., Papadopoulou, A., Anastasiadou, E., Baloyanni, N., Paronis, E., et al. (2011). Proteomics reveal rat hippocampal lateral asymmetry. Hippocampus 21, 108–119. doi: 10.1002/hipo.20727
Schmidt, M. F., Storrs, J. M., Freeman, K. B., Jack, C. R., Jr., Turner, S. T., Griswold, M. E., et al. (2018). A comparison of manual tracing and freesurfer for estimating hippocampal volume over the adult lifespan. Hum. Brain Mapp. 39, 2500–2513. doi: 10.1002/hbm.24017
Sowell, E. R., Peterson, B. S., Thompson, P. M., Welcome, S. E., Henkenius, A. L., and Toga, A. W. (2003). Mapping cortical change across the human life span. Nat. Neurosci. 6, 309–315. doi: 10.1038/nn1008
Sullivan, E. V., Pfefferbaum, A., Rohlfing, T., Baker, F. C., Padilla, M. L., and Colrain, I. M. (2011). Developmental change in regional brain structure over 7 months in early adolescence: comparison of approaches for longitudinal atlas-based parcellation. NeuroImage 57, 214–224. doi: 10.1016/j.neuroimage.2011.04.003
Suzuki, M., Hagino, H., Nohara, S., Zhou, S. Y., Kawasaki, Y., Takahashi, T., et al. (2005). Male-specific volume expansion of the humanhippocampus during adolescence. Cereb. Cortex 15, 187–193. doi: 10.1093/cercor/bhh121
Tamnes, C. K., Bos, M. G. N., van de Kamp, F. C., Peters, S., and Crone, E. A. (2018). Longitudinal development of hippocampal subregions from childhood to adulthood. Dev. Cogn. Neurosci. 30, 212–222. doi: 10.1016/j.dcn.2018.03.009
Tamnes, C. K., Walhovd, K. B., Dale, A. M., Østby, Y., Grydeland, H., Richardson, G., et al. (2013). Brain development and aging: overlapping and unique patterns of change. NeuroImage 68, 63–74. doi: 10.1016/j.neuroimage.2012.11.039
Tan, A., Ma, W., Vira, A., Marwha, D., and Eliot, L. (2016). The human hippocampus is not sexually-dimorphic: Meta-analysis of structural MRI volumes. NeuroImage 124, 350–366. doi: 10.1016/j.neuroimage.2015.08.050
Thal, D., Rub, U., Schultz, C., Sassin, I., Ghebremedhin, E., Del Tredici, K., et al. (2000). Sequence of Aβ-protein deposition in the human medial temporal lobe. J. Neuropathol. Exp. Neurol. 59, 733–748. doi: 10.1093/jnen/59.8.733
Voineskos, A. N., Winterburn, J. L., Felsky, D., Pipitone, J., Rajji, T. K., Mulsant, B. H., et al. (2015). Hippocampal (subfield) volume and shape in relation to cognitive performance across the adult lifespan. Hum. Brain Mapp. 36, 3020–3037. doi: 10.1002/hbm.22825
Wang, X., Yu, Y., Zhao, W., Li, Q., Li, X., Li, S., et al. (2018). Altered whole-brain structural covariance of the hippocampal subfields in subcortical vascular mild cognitive impairment and amnestic mild cognitive impairment patients. Front. Neurol. 9:342. doi: 10.3389/fneur.2018.00342
Wierenga, L. M., Langen, M., Ambrosino, S., van Dijk, S., Oranje, B., and Durston, S. (2014). Typical development of basal ganglia, hippocampus, amygdala and cerebellum from age 7 to 24. NeuroImage 96, 67–72. doi: 10.1016/j.neuroimage.2014.03.072
Yassa, M. A., and Stark, C. E. (2011). Pattern separation in the hippocampus. Trends Neurosci. 34, 515–525. doi: 10.1016/j.tins.2011.06.006
Keywords: hippocampus, development, MRI, gender, subregion
Citation: Mu SH, Yuan BK and Tan LH (2020) Effect of Gender on Development of Hippocampal Subregions From Childhood to Adulthood. Front. Hum. Neurosci. 14:611057. doi: 10.3389/fnhum.2020.611057
Received: 28 September 2020; Accepted: 09 November 2020;
Published: 03 December 2020.
Edited by:
Jiaojian Wang, University of Electronic Science and Technology of China, ChinaReviewed by:
Nizhuan Wang, Jiangsu Ocean Universiity, ChinaHong-Yan Bi, Chinese Academy of Sciences (CAS), China
Copyright © 2020 Mu, Yuan and Tan. This is an open-access article distributed under the terms of the Creative Commons Attribution License (CC BY). The use, distribution or reproduction in other forums is permitted, provided the original author(s) and the copyright owner(s) are credited and that the original publication in this journal is cited, in accordance with accepted academic practice. No use, distribution or reproduction is permitted which does not comply with these terms.
*Correspondence: Shu Hua Mu, shuhuamu@163.com; Li Hai Tan, tanlh@sions.cn