A Functional Near-Infrared Spectroscopy Examination of the Neural Correlates of Mental Rotation for Individuals With Different Depressive Tendencies
- 1Teachers College, Jimei University, Xiamen, China
- 2Institute of Special Environment Medicine, Nantong University, Nantong, China
- 3School of Foreign Languages, Jimei University, Xiamen, China
The present study aimed to examine the neural mechanisms underlying the ability to process the mental rotation with mirrored stimuli for different depressive tendencies with psychomotor retardation. Using functional near-infrared spectroscopy (fNIRS), we measured brain cortex activation of participants with higher and lower depressive tendencies while performing a left-right paradigm of object mental rotation or a same-different paradigm of subject mental rotation. Behavioral data revealed no differences in reaction time and rotation speed. The fNIRS data revealed a higher deactivation of oxyhemoglobin (HbO) change for the higher depression group in the perceptual stage of object mental rotation with mirrored stimuli in the superior external frontal cortex (BA46), inferior frontal gyrus (BA45), premotor cortex (BA6), and primary motor cortex (BA4) (study 1). In addition, there existed a significant difference between the two groups in premotor cortex (BA6) in subject mental rotation with mirrored stimuli (study 2). These results suggest that the neural mechanism of higher depression individuals connected with psychomotor retardation exists in the frontal and motor areas when processing object mental rotation with mirrored stimuli, and the motor cortex when processing subject mental rotation.
Introduction
Individuals with depression have difficulties in emotion and cognition, presenting depressive mood for more than 2 weeks, being anhedonia, being bias toward negative information, an inhibition disorder to information, and being psychomotor retardation. According to Beck’s Unified Model of Depression (Beck and Bredemeier, 2016), depression can be viewed as an adaptation to conserve energy after the perceived loss of an investment in a vital resource such as a relationship, group identity, or personal asset. The development of processing information negatively and biological reactivity to stress mediated by alterations in brain areas/networks involved in cognition and emotion regulation leads to the negative cognitive triad; in turn, the formation and activation of the negative cognitive triad further exacerbate cognitive biases and stress reactivity. In a sense, depression is an anachronistic manifestation of an evolutionarily based “depression program” (Beck and Bredemeier, 2016), which might be connected with a specific state of the brain cortex.
Individuals with depression disorder have some specific brain cortex. The prefrontal cortex (PFC) has emerged as one of the regions most consistently impaired in major depressive disorder (Pizzagalli and Roberts, 2021). Brain structures altered in depression are part of several connectivity networks, such as in the Default Mode Network (DMN) and the Cognitive Control Network (CCN) (Gudayol-Ferré et al., 2015). Yan et al. (2019) found reduced rather than increased functional connectivity within the default mode network for major depressive disorder. In a systematic review with 68 longitudinal neuroimaging studies using fMRI and EEG, blunted reward-related (striatal) activity was a potential biological marker for both onset and course of major depression disorder (Toenders et al., 2019). Region-specific fNIR leads show patients with unipolar depression had lower hemodynamic activation in the left dorsolateral prefrontal cortex (DLPFC), orbitofrontal cortex (OFC), bilateral ventrolateral prefrontal cortex (VLPFC), and left inferior frontal gyrus (IFG) relative to healthy population (Feng et al., 2021). Some studies on depression demonstrated deactivation. Deactivation of depressive patients was more apparent in the whole brain, especially in the cingulate gyrus using fMRI (Ma et al., 2009). Patients with bipolar disorder showed more deactivation in the medial prefrontal cortex than those with unipolar depression (Rodríguez-Cano et al., 2017). Therefore, the information processing of depressive person involves the frontal cortex, and the deactivation might be presented.
Psychomotor retardation is viewed as the core of the depressive syndrome (Lemke et al., 1999), which is often assessed by means of a specific clinical rating scale (Luca et al., 2013). Mental rotation has been extensively used to gain insight into the action system of clinical populations (Oshiyama et al., 2018), such as depression, because of the advantage of mental rotation investigating the internal processes of action planning and preparation and avoiding sensory and motor confounds related to motor execution. Psychomotor retardation of depression patients was not just a symptom of emotion but a potential neurophysiological defect (Austin et al., 1999), and was associated with damage of mental rotation (Rogers et al., 2002). Psychomotor retardation was a central feature of depression that can have clinical and therapeutic implications (Bennabi et al., 2014), and may strongly influence a depressive person’s psychosocial functioning. Relative to healthy controls, depressed patients showed a generalized motor slowing (Rogers et al., 2002; Bennabi et al., 2014), and more significant temporal discrepancies on both actual and mental movements (Bennabi et al., 2014), reflecting a role of declined motor planning and prediction in the psychomotor retardation of depression patients. Mental rotation might be one better way to investigate psychomotor retardation of depressive person, being superior to some rating scales.
Mental rotation is a cognitive function whereby objects, images, or the body are mentally imaged and rotated through 2-Dimension or 3-Dimension (Shepard and Metzler, 1971). Mental rotation is a spatial representational capability of imaging or mentally rotating an object or subject. It enables one to judge contexts through viewing objects from rotated angles. Some researchers provided some arguments about the processing stages of mental rotation, including a 2-stage (Jansen et al., 2012), 3-stage (Just and Carpenter, 1976; Yan et al., 2013), and 5-stage (Corballis, 1988). Based on information processing theory and events related potentials (ERPs), Yan et al. (2013) proposed that cognitive processing of mental rotation has a perceptual stage, rotation stage and decision stage in turn. Ke and Wang (2021) found the novice drivers performed differently at perceptual and rotation stages in mental rotation about driving task. Taking superiority of processing into consideration, Jansen et al. (2012) took the reaction time in an un-rotation stage as an index of the perceptual stage, viz., the rotating angle is 0, and took the rotating speed as an index of rotation stage, viz., angle divided by corresponding reaction time. Jansen’s viewpoints have been supported by some studies (Ozel et al., 2002; Jansen et al., 2012; Feng et al., 2019; Ke and Wang, 2021), supporting that the rotating speed is a better index over the correct rate. Neuroimaging studies on healthy populations revealed activation of specific brain regions recruited spatial cognitive processing, such as frontal (BA for Brodmann Area, BA9, BA10), premotor (BA6), parietal cortex (BA40, BA44) (Hyun and Luck, 2007). Further, the areas of activation in mental rotation were premotor area, bilateral superior parietal lobule and visual extrastriate cortex (Vingerhoets et al., 2002). Some neuroscience studies have identified brain regions activated in the frontal cortex (BA9, BA44), premotor cortex (BA6) and parietal cortex (BA40), basal ganglia, cerebellum during mental rotation (Jordan et al., 2001; Schöning et al., 2007). Using fNIRS, increased oxyhemoglobin (HbO) in BA44 was observed in high and low-performance groups. In contrast, a decrease in deoxyhemoglobin (HbR) in BA9 was observed in only the high-performance group and BA44 only in the low-performance group. The BA44 is considered one of the core neural correlates of mental rotation, while BA6 and BA9 might be compensatory (Wu et al., 2020). Thus, the frontal cortex and motor cortex might participate in the mental rotation processing, especially involving the BA6, BA9, BA40, and BA44.
Mental rotation has object and subject mental rotation according to the stimuli type of task, with mirrored or identical (normal) stimuli. An object, such as letter, digit, 3-D picture and true scene, or subject, such as body of person and body parts (hand, arm, leg, and face), is mentally rotated in mental rotation, referring to object mental rotation or subject mental rotation, respectively (Dalecki et al., 2012; Chen and Hu, 2019). Chen et al. (2013a) found mental rotation deficits specific to the hand than the letter task, suggesting that the mental imagery for hands and letters relies on different processing mechanisms. Compared to the healthy controls, depression patients demonstrated a lower error rate, shorter reaction time and larger P500 aptitude in mirror image mental rotation, reflecting an impairment of information processing of representation rotating in patients with depressive disorder (Chen et al., 2013b,c). Furthermore, some ERPs studies showed that mirror-normal difference in both the early and late phases of mental rotation and deduced that flipping is more likely to occur in the late phase of the mirror-normal letter discrimination task (Quan et al., 2017), and hypoxia effect was effective with normal letters but had little effect on the mirrored letters (Ma et al., 2016). A Beta-band ERD Study found the temporal difference of beta ERD between the identical and the mirrored stimuli at 0°rotation and the ERD topographic difference in left fronto-parietal regions (Chen et al., 2014). A self-paced event-related fMRI design revealed the differences in visual cortex activation between tasks with mirrored and identical figures (Paschke et al., 2012). So, processing deficits related to object mental rotation with depression might be different from that of subject mental rotation, and it is necessary to differentiate mirrored from identical tasks in mental rotation experiment.
Usually, different experiment paradigms are employed to different kinds of mental rotation. A left-right paradigm (L-R paradigm) is often conducted in object mental rotation in which the original and mirrored images are simultaneously set on the left and right parts of the screen, respectively, and participants are required to judge the similarity of two images; a same-different paradigm (S-D paradigm) is used in subject mental rotation in which two kinds of stimuli present separately in the order, and participants are asked to judge whether the target stimulus is the same as the original stimulus stayed in the short memory (Jolicocur et al., 1987; Kosslyn et al., 2001; Dalecki et al., 2012; Chen and Hu, 2019). The nature of image stimulus in the mental rotation might be the key to lead to the activation of motor area; when the stimulus was subject, the motor cortex (M1) was activated, and premotor cortex was also activated for half of participants (Kosslyn et al., 1998, 2001).
Relative to the other techniques including EEG, fMRI, and PET, fNIRS has some advantages. fNIRS is a non-invasive optical imaging technique that measures changes in hemoglobin (Hb) concentrations within the brain by means of the characteristic absorption spectra of Hb in the near-infrared range. fNIRS systems are relatively insensitive to participant motion and have a portable, compact, and increasingly miniaturized design (Di Domenico et al., 2019). Many studies support the reliability of fNIRS to measure the cerebral hemodynamics in spatial cognitive tasks (Herff et al., 2013; Fu et al., 2016). Unlike EEG or ERPs being heavily dependent on the superposed average of data, fNIRS focuses the data during a period of time. Therefore, relatively small sample size (Li et al., 2019; Mauri et al., 2020; Zhang et al., 2020), and relatively small trial size could be acceptable (Du et al., 2015). The fNIRS itself provides a better way to ascertain the processing of psychomotor retardation of depression in mental rotation, being better than scales or surveys.
The current study aimed to examine the relevant activation regions recruited mental rotation with mirrored stimuli for depressive tendencies individuals with psychomotor retardation. Here are two research questions. The research question 1 is that, is there differences of activation regions, mainly frontal and motor cortex, between different depressive tendencies in mental rotation. The research question 2 is that, is there any differences of activation regions above mentioned between object and subject mental rotation. Two experiments were conducted through fNIRS technique and a left-right paradigm (study 1) or a same-different paradigm (study 2) of mental rotation, in order to answer these two research questions. One factor between-subjects design was employed (Yan et al., 2021), with depressive tendency as independent variable. Two experiments were object mental rotation (Study 1) and subject mental rotation (Study 2), using mirrored stimuli, taking reaction time (Rt) at angle 0° as behavior index of the perceptual stage (stimulus encoding) and rotating speed as an index of the rotating stage (responsive to motor speed), taking beta of HbO change in brain areas as an index of biology. Taking the angle of rotation and reaction time into consideration, rotating speed is a sensitive index in measuring and assessing mental rotation (Ozel et al., 2002; Jansen et al., 2012; Feng et al., 2019; Ke and Wang, 2021). Based on the literature of mental rotation and depression patients, the overlapping cortex lies in frontal and motor cortex, the hypothesis 1 corresponding to the research question 1 is that, the differences of activation regions between different depressive tendencies in mental rotation could exists in frontal and motor cortex. According to the literature of object and subject mental rotation, the hypothesis 2 corresponding to the research question 2 is that, there would exist difference of activation regions above mentioned between object and subject mental rotation for depressive individuals.
Study 1
This experiment investigated the difference in activation areas recruited mirror movement in object mirror mental rotation between different depressive tendencies.
Method
Participants
A total of 41 college students participated in the experiment. All were right-handed, reported no brain or psychology illness history, normal version or corrected-version. Forty participants were analyzed, aged mean 23.63, SD = 1.90, one participant was excluded whose fNIRS signal record failed.
Materials and Tools
Object mirror images were GPS map by Photoshop2018 software, referred to map elements (Mei, 2011), and Baidu map (see Figure 1). These mirror images were generated at a spatial rotation angle of 0°, 90°, 180°, 270°, of them, 0° belonging to the perceptual stage, 90°, 180°, and 270° belonging to rotation stage.
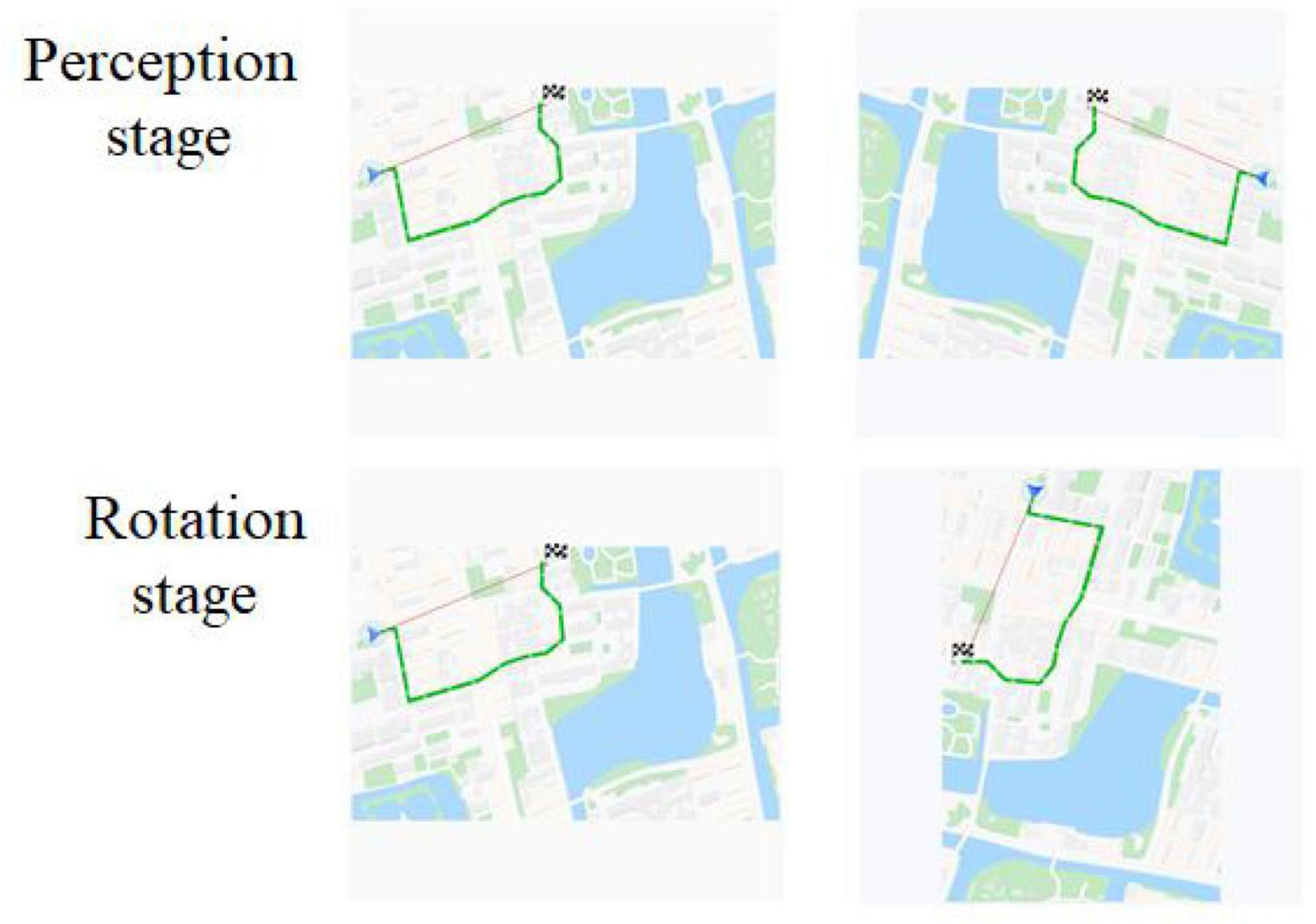
Figure 1. Experimental Materials for object mental rotation. The first column was original images, and the second column was rotation or mirror images.
BDI-II-C (Beck Depression Inventory, BDI) was a Chinese version of Beck Depression Inventory (Beck et al., 1996; Wang et al., 2011; Yang et al., 2014), applied to self-assess depression symptoms in the past 2 weeks, including 21 items.
Procedure
A left-right paradigm (L-R paradigm) was employed for this object mental rotation. The procedure was programmed with E-prime 3.0 software and presented on a 19-inch computer. Screen resolution was 1,440 × 900, and the refresh rate was 75 Hz. Before the experiment, the participant wore a fiber cap, and the equipment was calibrated. In addition, a 3-D head model was constructed. The procedure was as followed (Figure 2). After the instruction, the fixation “+” was on the middle of the screen for 800 ms, followed by two images on the left and right parts of the screen. The participant was required to judge whether these two images were the same if the images were rotated, pressed key “F” for the same, pressed key “J” for the difference. The style of key-pressed was counterbalanced. There were 48 trials (12 blocks), of which 24 trials were mirror images. The rest time for every block was 20 s.
Collecting and Analysis of fNIRS Data
The equipment of fNIRS with LABNIRS system was from Japan Shimadzu corporation, recording the concentration changes of oxygenated hemoglobin (HbO). There were 16 optrodes set in 10–20 systems, and there were 20 channels (Figure 3 and Table 1). 3D-digitizer positioned the coordinates of all channels and the areas of the brain include: (a) dorsolateral prefrontal cortex (DLPFC and BA9) corresponding to channels 2, 5, and 9; (b) superior external frontal cortex (BA46) corresponding to channels 1; (c) premotor cortex (BA6 and BA8) corresponding to channels 3, 6, 7, 10, 11, 12, 15, 16, and 20; (d) inferior frontal gyrus (IFG, BA44, and BA45) corresponding to channels 4, 8, 13, and 17; and (e) the primary motor cortex (BA4) corresponding to channels 14, 18, and 19.
Data of fNIRS were analyzed using NIRS_SPM software in Matlab (R2014b), which usually run based on a general linear model (GLM) (Ye et al., 2009). The current study mainly analyzed HbO, the most sensitive parameter to local brain blood and correlated to blood signals. The procedure of data analysis was as follows. Step 1 dealt with the noise elimination of raw data using the hemodynamic response function (HRF) and wavelet-minimum description length (wavelet-MDL). Step 2, parameter estimation, was taken through a GLM model to get beta value under every condition. Then, the beta was baseline-corrected in which ΔHbO2 of each channel was calculated as the difference between the beta of task-stage and the beta of baseline after 15 s. The positive beta meant activation, while minus beta meant deactivation (Rodrigo et al., 2014). Both were the state of brain activation. Step 3, the beta was analyzed and corrected using false discovery rate (FDR). FDR is usually used to correct the p-values of fNIRS data (Benjamini and Hochberg, 1995; Singh and Dan, 2006; Bai et al., 2016; Zhang et al., 2019).
Results
The raw score of BDI-II-C was transferred into a Z score. Twenty-seven participants were assigned to the lower depression tendencies group whose Z score was less than 0, while 13 participants were in the higher group whose Z score was greater than 0. The independent samples t-test showed that the raw score of BDI in the higher group (M = 11.38, SD = 7.01) was significantly greater than that in the lower group (M = 1.74, SD = 1.56), t(38) = 6.895, p < 0.001. Taking reaction time at angle 0° (non-rotation) as behavior index of the perceptual stage (stimulus encoding), and rotating speed as an index of the rotating stage which was mean of a ratio of angle and corresponding reaction time (Jansen et al., 2012), viz., the formula was, rotation speed .
The independent samples t-test showed no significant difference in the reaction time of the perceptual stage and rotation speed of the rotation stage (upper part of Table 2), ts < 1.
The independent samples t-test was conducted to examine the differences of beta values of HbO change in different channels in perceptual stage (Table 3), rotation stage (Table 4), between lower and higher groups. The results showed, HbO change in the higher depression group was smaller than that in the lower group in five channels, including channel 1 [t(38) = 3.013, d = 1.02, p < 0.01], channel 4 [t(38) = 2.813, d = 0.95, p < 0.01], channel 7 [t(38) = 3.174, d = 1.07, p < 0.01], channel 11 [t(38) = 2.157, d = 0.73, p < 0.05], and channel 14 [t(38) = 2.034, d = 0.69, p < 0.05], in perceptual stage.
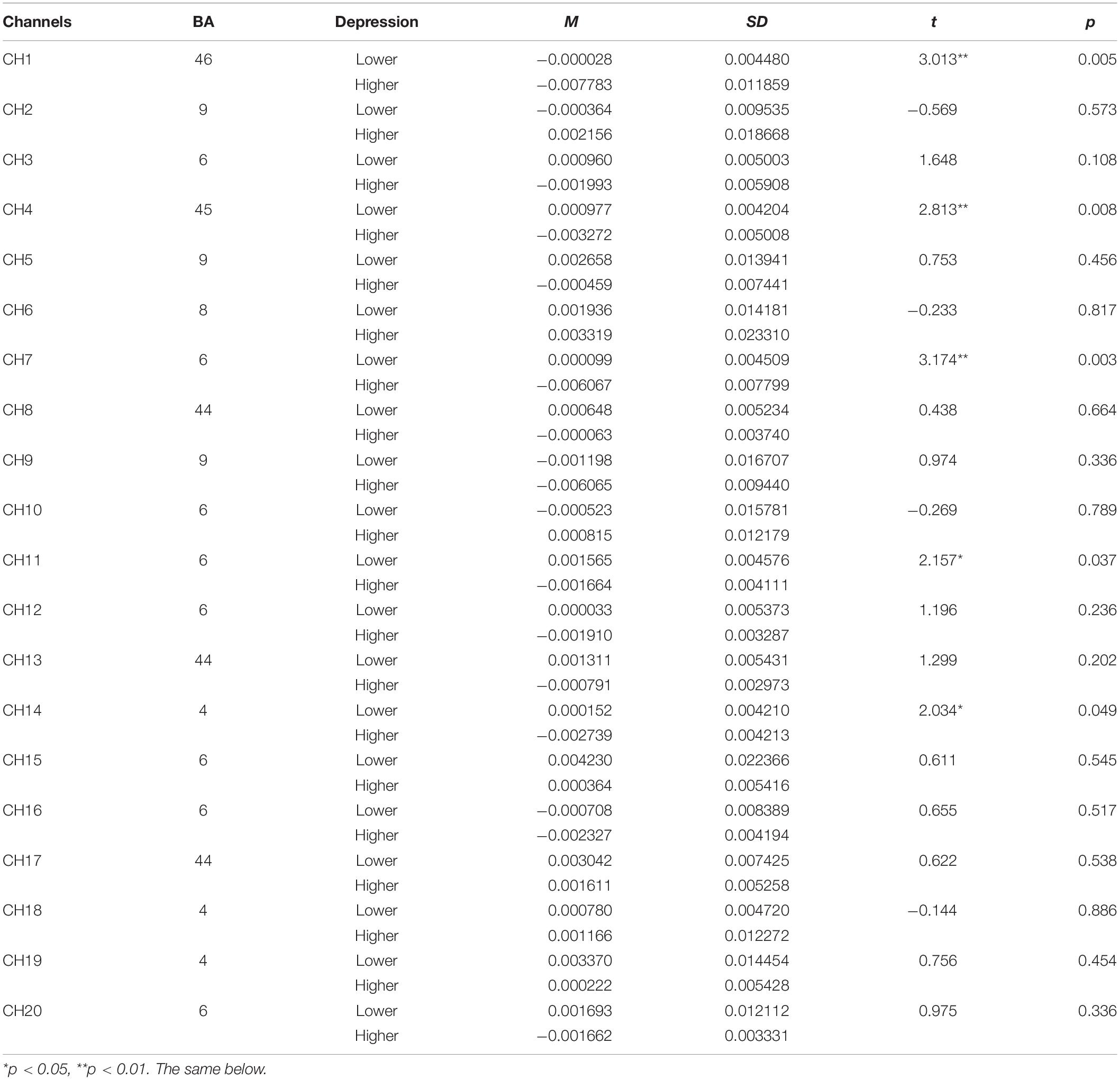
Table 3. The comparison of brain activation between higher and lower groups in perceptual stage for object mirrored images (HbO).
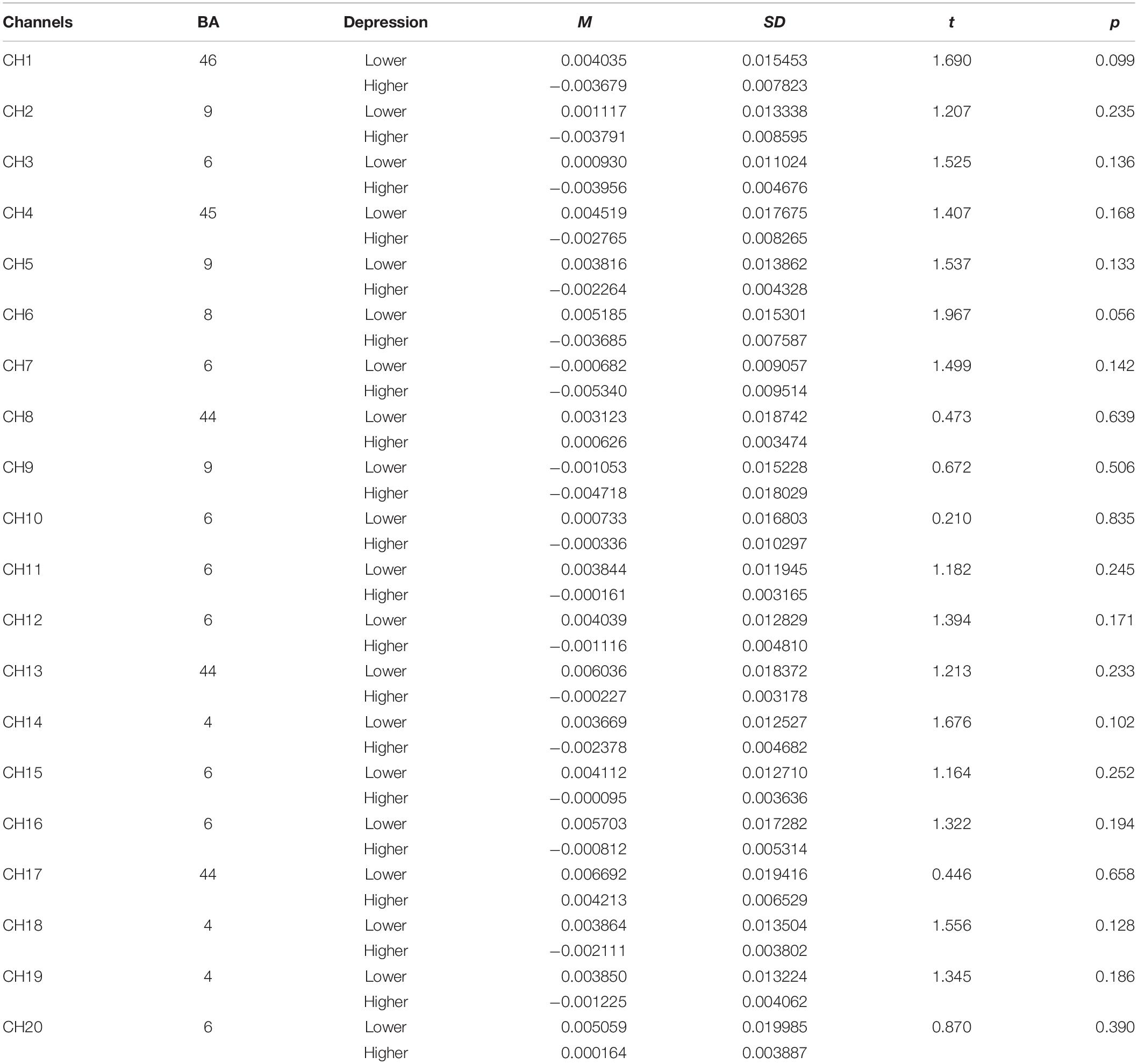
Table 4. The comparison of brain activation between higher and lower groups in rotation stage for object mirrored images (HbO).
The brain activation map of higher and lower groups was drawn based on t-values of channels (upper part of Figure 4). Specifically, the heat map of t-values of object mirror images in the perception stage covered channels 1, 4, 7, 11, and 14, corresponding to the superior external frontal cortex (BA46), inferior frontal gyrus (BA45), premotor cortex (BA6), the primary motor cortex (BA4), indicating a more substantial deactivation of the higher group.
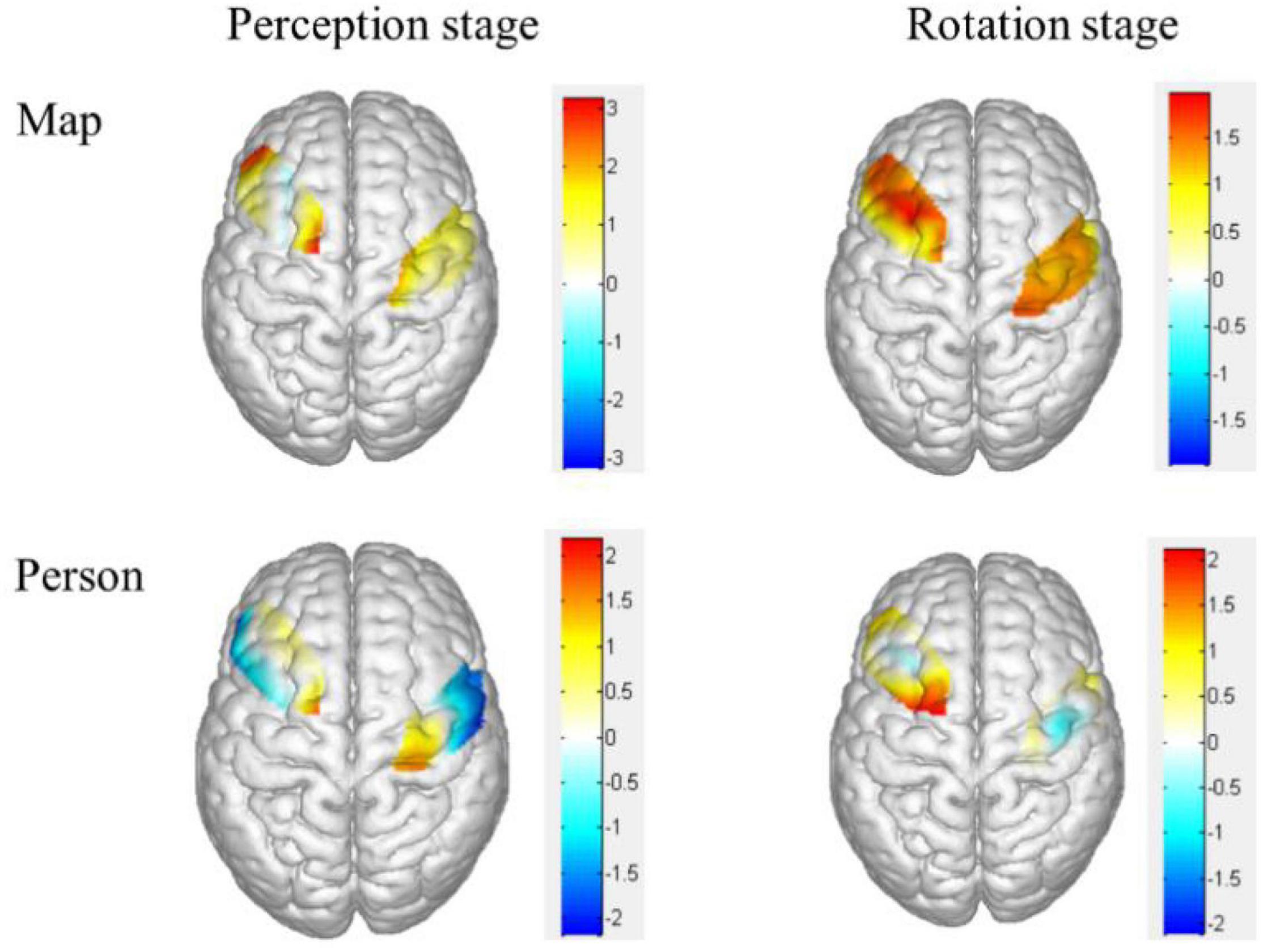
Figure 4. Heat map of t-values for the comparison of different groups of brain activation in different stages. The upper was the object mirror images, and the bottom was the subject mirror images. The bigger the two groups, the thicker the color.
Discussion
The experiment of study 1 found the deactivation areas recruited in object mental rotation with mirrored stimuli for higher depressive tendencies group are located in the superior external frontal cortex (BA46), inferior frontal gyrus (BA45), premotor cortex (BA6), the primary motor cortex (BA4), relative to lower group, indicating the frontal and motor areas related with psychomotor retardation of depression. These two areas execute the mental rotation with psychomotor retardation, co-functioning the inhibition and motor.
Study 2
This experiment investigated the difference in activation areas recruited mirror movement in subject mental rotation between different depressive tendencies.
Method
Participants, tools, procedure, method of fNIRS data collecting and analysis in study 2 were the same as those in study 1. Materials were subject images of traffic police taking eight gestures (Figure 5). Person images were rotated at angles of 0°, 30°, 60°, and 90°. A same-different paradigm (S-D paradigm) was employed for this subject mental rotation. The method of stimuli presented in S-D paradigm was different from the L-R paradigm of study 1, in which the images of original image was presented before, then the rotation or mirrored image came out in S-D paradigm (Figure 6).
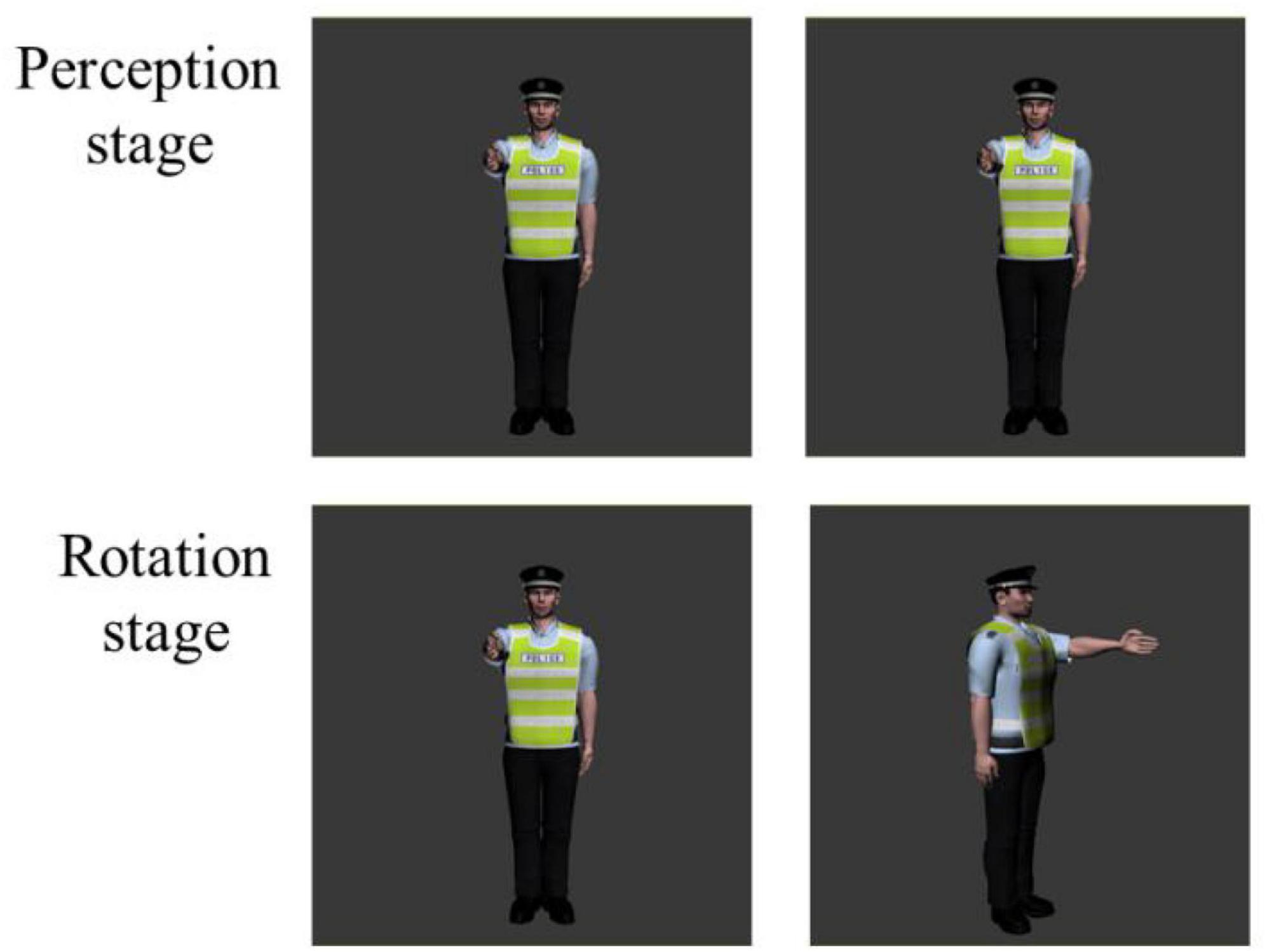
Figure 5. Experimental Materials for subject mental rotation. The first column were original images, and the second column were rotation or mirrored images.
Results
Regarding the behavior data, the independent samples t-test showed no significant difference in reaction time of the perceptual stage and rotation speed of the rotation stage (see Table 2), ts < 1.
Concerning the fNIRS data, the independent samples t-test was conducted to examine the differences of beta values in different channels in perceptual stage (Table 5), rotation stage (Table 6), between lower and higher groups. The results showed, changes of oxygenated hemoglobin (HbO) in higher depression group was bigger than that in lower group in channel 20 in perceptual stage [t(38) = -2.178, d = 0.74, p < 0.05], and smaller in the rotation stage in channel 7 [t(38) = 2.106, d = 0.71, p < 0.05] and channel 10 [t(38) = 2.089, d = 0.55, p < 0.05].
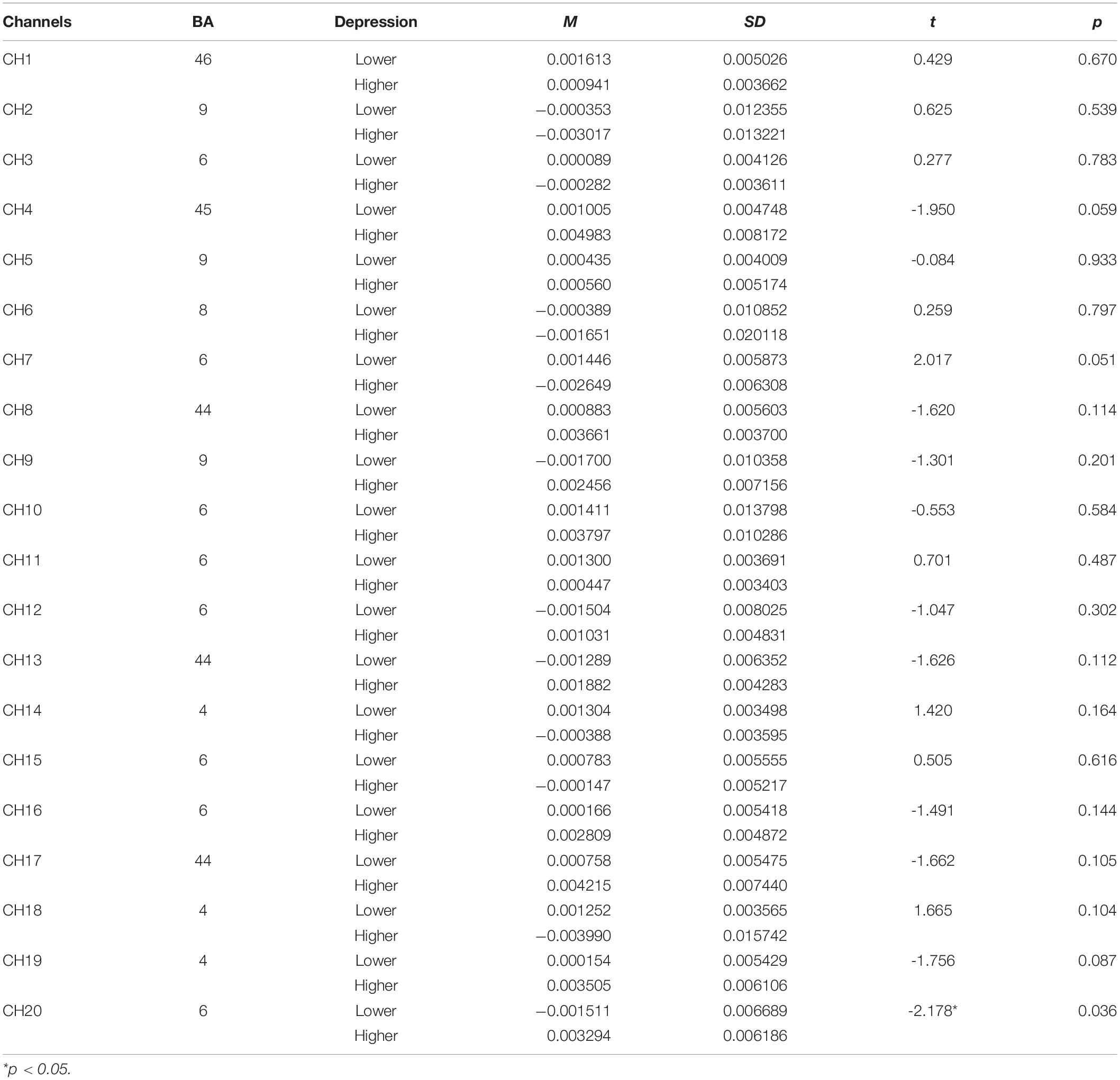
Table 5. The comparison of brain activation between higher and lower groups in perceptual stage for subject mirrored images (HbO).
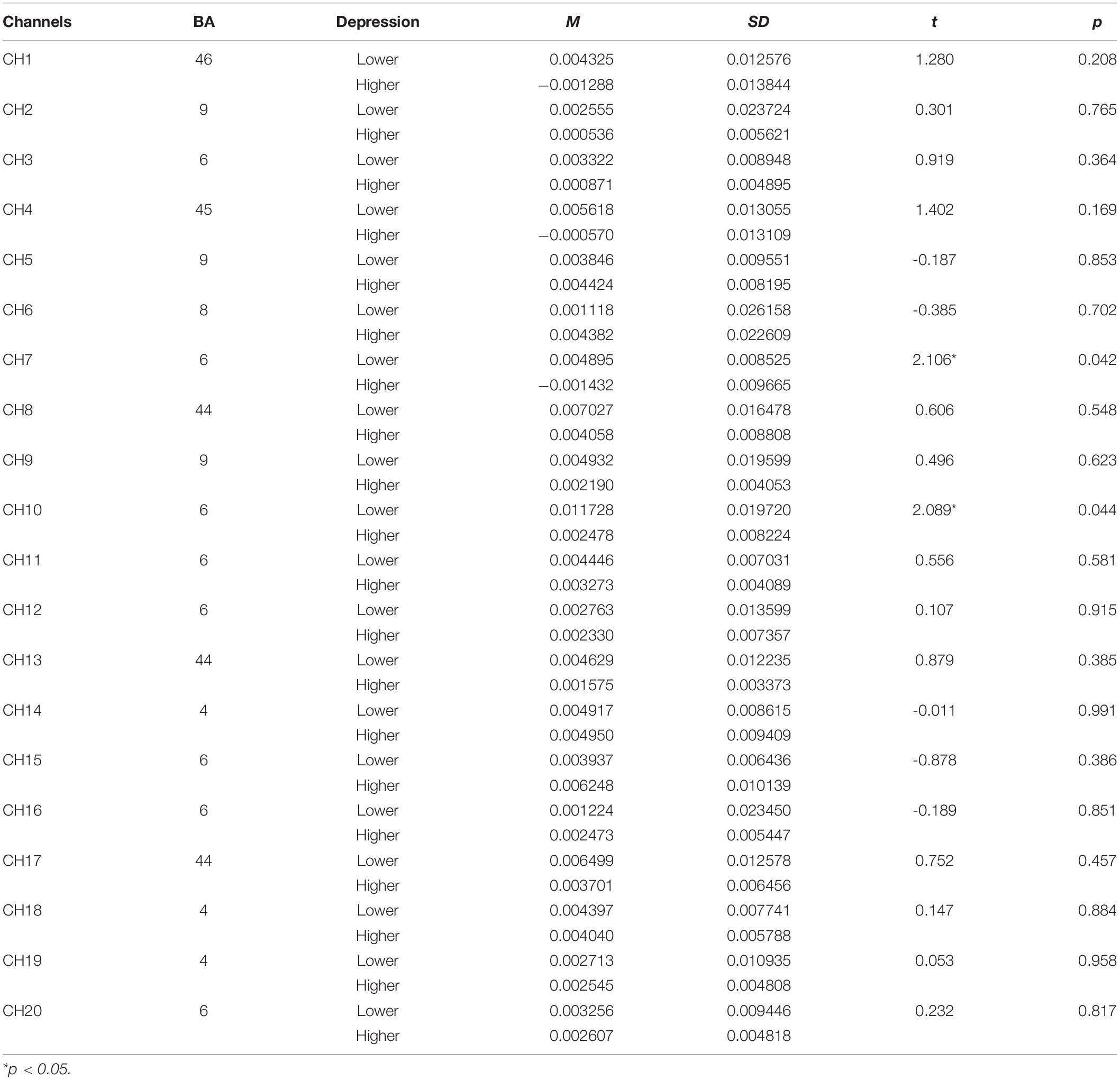
Table 6. The comparison of brain activation between higher and lower groups in rotation stage for subject mirrored images (HbO).
The brain activation map of higher and lower groups was drawn based on t-values of channels (bottom part of Figure 4). The t-values hot contrast images of subject mirror images in the perception stage covered channel 20 corresponding to the premotor cortex (BA6), in rotation stage channel 7 and 10 corresponding to the premotor cortex (BA6).
Discussion
The experiment of study 2 found the deactivation area recruited in subject mental rotation with mirrored stimuli for higher depressive tendencies group lies in the premotor cortex (BA6) in perceptual stage, and the activation in the premotor cortex (BA6) in rotation stage, relative to lower group, indicating only motor areas related with psychomotor retardation of depression. It is different from the results of object mental rotation in study 1 in which the frontal and motor cortex were recruited for higher group. Subject mental rotation only involves the premotor cortex (BA6).
General Discussion
This research mainly found a higher deactivation of changes of oxygenated hemoglobin (HbO) for higher depressive tendency participants in object mental rotation with mirrored stimuli in the superior external frontal cortex (BA46), inferior frontal gyrus (BA45), premotor cortex (BA6), the primary motor cortex (BA4), indicating a unique role of the frontal cortex and motor cortex in mental rotation processing of depression individual. These findings could correspond the research question 1 and evidences the hypothesis 1. Mental rotation is a spatial representation located in the functional areas, including the premotor cortex and primary motor cortex (Jordan et al., 2001; Vingerhoets et al., 2002; Hyun and Luck, 2007; Hétu et al., 2013). The deactivation of the frontal cortex is recruited in spatial cognitive processing for depression patients (Hyun and Luck, 2007; Rodríguez-Cano et al., 2017; Feng et al., 2021), which is consistent with the founding of the current study. Psychomotor retardation of depression associated with damage of mental rotation (Austin et al., 1999; Rogers et al., 2002), did not come out by way of an explicit index, such as reaction time and rotation speed in this study, supporting “depression program” in Beck’s Unified Model of Depression (Beck and Bredemeier, 2016).
Deactivation is the metabolism attenuation of cerebral blood flow during cognitive task processing. It is unconscious and cannot be aware. That is to say, significant increases in oxyhemoglobin (HbO) compared to baseline were observed in the task of processing mental rotation, and brain blood flow, level of HbO and local brain nerve activity would attenuate. The function of the deactivation area is to focus on the attention and to allocate the attention resource automatically. Deactivation of the corresponding brain area would come out, when individuals pay attention to execute one task which reduces the activity of extensive information collection, and the level of activation lowers down (Feng et al., 2007; Li and Shu, 2014). Deactivation might function as inhibition or monitoring of some information in which some redundancy information can be filtered to amplify useful information (Drevets et al., 1995). The frontal cortex is a default mode network connected with control of cognition and monitoring tasks, especially in representing target maintenance and inhibition (Paxton et al., 2008; Yan et al., 2019). Prefrontal cortex deactivation means a control strategy to cognitive resources to represent cue information (Zhang et al., 2020). Some fNIRS studies reported deactivation of dorsolateral prefrontal cortex (DLPFC) and ventrolateral prefrontal cortex (VLPFC) during inhibition control (Nishimura et al., 2011; Wriessnegger et al., 2012; Rodrigo et al., 2014). When depressive individuals process mental rotation, cognitive resources need to reassign, leading to a bigger HbO change, consistent with the findings by Shmuel et al. (2002).
Another finding is the difference between object and subject mental rotation with mirrored stimuli for higher depressive tendency individuals, corresponding to the research question 2 and supporting the hypothesis 2, indicating a different processing mechanism between object and subject mental rotation for depressive individuals. Compared to the lower depressive group, the higher group recruits the frontal cortex to process the object mental rotation relative to subject mental rotation, including superior external frontal cortex (BA46) and inferior frontal gyrus (BA45). This result is in line with one mental rotation study of healthy population (Vingerhoets et al., 2002). One explanation is about the stimuli types in different paradigms of object and subject mental rotation which affect the spatial representation (Kosslyn et al., 1998, 2001). The task of L-R paradigm in object mental rotation (i.e., study 1) includes the searching, transforming, comparing and confirming (Shepard and Metzler, 1971), the task of S-D paradigm in subject mental rotation (i.e., study 2) mainly focuses judging rotation (Jolicocur et al., 1987). In a sense, the former requires more monitoring and translation which are function of frontal cortex, comparing with the latter. Alternative explanation is about embodied cognition. The activation of cortical regions during mental rotation seems at least in part determined by an intrinsic process that depends on the afforded actions elicited by the kind of stimuli presented. More studies of healthy population using ERP, ERD, or fMRI techniques found a different neural mechanism between object and subject mental rotation (Paschke et al., 2012; Chen et al., 2014; Ma et al., 2016; Quan et al., 2017). Further, Chen et al. (2013b,c) reported that patients with depressive disorder had an impairment processing mental rotation with mirrored stimuli. In the perceptual stage of mental rotation with mirrored stimuli, participants need to imagine themselves in different angles, embodied themselves in the object (map) or subject (person) position. A study on a healthy population demonstrates a more vital link between the bodily self and motor representations (Kaltner et al., 2014). The extent of embodiment in an object is more complex than that in a subject (the body of a person) (Barsalou, 2008; Wang and Sang, 2014). Using human bodies as stimulus material elicits embodied spatial transformations (Kaltner et al., 2014). The human body (i.e., traffic policeman in study 2), not object (i.e., map in study 1), induces embodied spatial representation. Thus, the specific brain area of monitoring and inhibition, i.e., frontal brain areas, would be recruited for participants of the higher group to execute the object mental rotation.
Other, there were no significant differences between higher and lower groups in the reaction time of the perceptual stage and rotation speed of the rotation stage in both object and subject mental rotation. It seems that the results of these behavioral indices are not consistent with the results of fNIRS data. Here is one explanation from the perspective of nature of two kinds of measure data. Behavioral data focus the behavioral results of whole processing which includes the different stages of processing. The fNIRS data focus the brain tissue concentration changes in oxyhemoglobin (HbO2) and deoxyhemoglobin (HbR) associated with an increased metabolic demand of the brain during neuronal activity (Pinti et al., 2019), presented during a certain time period. The latter is more accurate and is out of the control of consciousness.
In sum, our work was the first study to uncover the underlying neural mechanisms of psychomotor retardation of depressive individuals measured by mental rotation using the fNIRS method. It is concluded that the neural areas of higher depressive tendency individuals connected with psychomotor retardation exists in the frontal and motor cortex when processing object mental rotation, and the motor cortex when processing subject mental rotation.
Data Availability Statement
The original contributions presented in the study are included in the article/Supplementary Material, further inquiries can be directed to the corresponding author.
Ethics Statement
The studies involving human participants were reviewed and approved by the Nantong University Committee. The patients/participants provided their written informed consent to participate in this study.
Author Contributions
LW and HZ developed the study concept, contributed to the design, and wrote and revised the manuscript. LW and JK implemented the experiment, and collected and analyzed the data. All authors contributed to the article and approved the submitted version.
Funding
This study was funded by the National Social Science Foundation of China (20BYY071).
Conflict of Interest
The authors declare that the research was conducted in the absence of any commercial or financial relationships that could be construed as a potential conflict of interest.
Publisher’s Note
All claims expressed in this article are solely those of the authors and do not necessarily represent those of their affiliated organizations, or those of the publisher, the editors and the reviewers. Any product that may be evaluated in this article, or claim that may be made by its manufacturer, is not guaranteed or endorsed by the publisher.
Supplementary Material
The Supplementary Material for this article can be found online at: https://www.frontiersin.org/articles/10.3389/fnhum.2022.760738/full#supplementary-material
References
Austin, M. P., Mitchell, P., Wilhelm, K., Parker, G., Hickie, I., Brodaty, H., et al. (1999). Cognitive function in depression: a distinct pattern of frontal impairment in melancholia? Psychol. Med. 29, 73–85. doi: 10.1017/S0033291798007788
Bai, X., Zhang, Q., Zhang, P., Zhou, S., Liu, Y., Song, X., et al. (2016). Comparison of motor execution and motor imagery brain activation patterns: A fNIRS study (in Chinese). Acta Psychol. Sinica 48, 495–508. doi: 10.3724/SP.J.1041.2016.00495
Barsalou, L. W. (2008). Grounded cognition. Ann. Rev. Psychol. 59, 617–645. doi: 10.1146/annurev.psych.59.103006.093639
Beck, A. T., and Bredemeier, K. (2016). A unified model of depression: Integrating clinical, cognitive, biological, and evolutionary perspectives. Clin. Psychol. Sci. 4, 596–619. doi: 10.1177/2167702616628523
Beck, A. T., Steer, R. A., and Brown, G. K. (1996). Manual for the Beck Depression Inventory-II. San Antonio, TX: Psychological Corporation.
Benjamini, Y., and Hochberg, Y. (1995). Controlling the false discovery rate: a practical and powerful approach to multiple testing. J. Royal Stat. Soc. Ser. B 57, 289–300. doi: 10.1111/j.2517-6161.1995.tb02031.x
Bennabi, D., Monnin, J., Haffen, E., Carvalho, N., Vandel, P., Pozzo, T., et al. (2014). Motor imagery in unipolar major depression. Front. Behav. Neurosci. 8:413. doi: 10.3389/fnbeh.2014.00413
Chen, H., Guo, X., Lv, Y., Sun, J., and Tong, S. (2014). Mental rotation process for mirrored and identical stimuli: A beta-band ERD study. Annu. Int. Conf. IEEE Eng. Med. Biol. Soc. 2014, 4948–4951. doi: 10.1109/EMBC.2014.6944734
Chen, J., and Hu, Q. (2019). The cognitive and neural mechanism of body-part mental rotation (in Chinese). Psychol. Dev. Educ. 35, 376–384. doi: 10.16187/j.cnki.issn1001-4918.2019.03.16
Chen, J., Yang, L., Ma, W., Wu, X., Zhang, Y., Wei, D., et al. (2013a). Ego-rotation and object-rotation in major depressive disorder. Psychiatry Res. 209, 32–39. doi: 10.1016/j.psychres.2012.10.003
Chen, J., Yang, L., Wu, X., Ma, W., Liu, G., Deng, Z., et al. (2013b). Effect of aging on information processing of representation rotating in patients with major depressive disorder (in Chinese). Chin. J. Neuromed. 112, 511–515. doi: 10.3760/cmad.issn.1671-8925.2013.05.017
Chen, J., Yang, L., Wu, X., Ma, W., Zhang, Y., Liu, G., et al. (2013c). Mental rotation evoked potentials P500 in patients with major depressive disorder (in Chinese). Chin. J. Psychiatry 46, 78–84. doi: 10.3760/cma.j.issn.1006-7884.2013.02.003
Corballis, M. C. (1988). Recognition of disoriented shapes. Psychol. Rev. 95, 115–123. doi: 10.1037/0033-295X.95.1.115
Dalecki, M., Hoffmann, U., and Bock, O. (2012). Mental rotation of letters, body parts and complex scenes: Separate or common mechanisms? Human Movem. Sci. 31, 1151–1160. doi: 10.1016/j.humov.2011.12.001
Di Domenico, S. I., Rodrigo, A. H., Dong, M., Fournier, M. A., Ayaz, H., Ryan, R. M., et al. (2019). “Functional Near-Infrared Spectroscopy: Proof of Concept for Its Application in Social Neuroscience,” in Neuroergonomics: The Brain at Work and in Everyday Life, eds H. Ayaz and F. Dehais (Amsterdam: Elsevier), 169–173. doi: 10.1016/C2016-0-02196-4
Drevets, W. C., Burton, H., Videen, T. O., Snyder, A. Z., Simpson, J. R., and Raichle, M. E. (1995). Blood flow changes in human somatosensory cortex during anticipated stimulation. Nature 373, 249–252. doi: 10.1038/373249a0
Du, K., Wang, L., Feng, Z., Zhang, Y., and Qiu, M. (2015). Brain activation modes during motor execution and motor imagery in healthy individuals by functional near infrared reflectance spectroscopy (in Chinese). J. Third Milit. Med. Univ. 37, 2017–2021. doi: 10.16016/j.1000-5404.201503179
Feng, H., Zhang, Y., Tang, Y., Jin, J., Dong, F., Feng, S., et al. (2007). Deactivation of brain in the digit-sum task (in Chinese). Chin. Sci. Bullet. 52, 1280–1284. doi: 10.3321/j.issn:0023-074X.2007.11.011
Feng, K., Law, S., Ravindran, N., Chen, G., Ma, X., Bo, X., et al. (2021). Differentiating between bipolar and unipolar depression using prefrontal activation patterns: Promising results from functional near infrared spectroscopy (fNIRS) findings. J. Affect. Disord. 281, 476–484. doi: 10.1016/j.jad.2020.12.048
Feng, T., Li, Y., Ji, Z., and Zhang, Z. (2019). The Role of Time constraints in athletes’ egocentric mental rotation performance. Adv. Cogn. Psychol. 15, 225–235. doi: 10.5709/acp-0270-6
Fu, G., Wan, N. J. A., Baker, J. M., Montgomery, J. W., Evans, J. L., and Gillam, R. B. (2016). A proof of concept study of function-based statistical analysis of fNIRS data: Syntax comprehension in children with specific language impairment compared to typically-developing controls. Front. Behav. Neurosci. 10:108. doi: 10.3389/fnbeh.2016.00108
Gudayol-Ferré, E., Peró-Cebollero, M., González-Garrido, A. A., and Guàrdia-Olmos, J. (2015). Changes in brain connectivity related to the treatment of depression measured through fMRI: a systematic review. Front. Human Neurosci. 9:582. doi: 10.3389/fnhum.2015.00582
Herff, C., Heger, D., Putze, F., Hennrich, J., Fortmann, O., and Schultz, T. (2013). Classification of mental tasks in the prefrontal cortex using fNIRS. Annu Int Conf IEEE Eng Med Biol Soc 2013, 2160–2163. doi: 10.1109/EMBC.2013.6609962
Hétu, S., Grégoire, M., Saimpont, A., Coll, M., Eugène, F., Michon, P., et al. (2013). The neural network of motor imagery: An ALE meta-analysis. Neurosci. Biobehav. Rev. 37, 930–949. doi: 10.1016/j.neubiorev.2013.03.017
Hyun, J. S., and Luck, S. J. (2007). Visual working memory as the substrate for mental rotation. Psychon. Bull. Rev. 14, 154–158. doi: 10.3758/BF03194043
Jansen, P., Lehmann, J., and Van Doren, J. (2012). Mental rotation performance in male soccer players. PLoS One 7:e48620. doi: 10.1371/journal.pone.0048620
Jolicocur, P., Snow, D., and Murray, J. (1987). The time to identity disoriented letters: effects of practice and font. Can. J. Psychol. 41, 303–316. doi: 10.1037/h0084159
Jordan, K., Heinze, H. J., Lutz, K., Kanowski, M., and Jäncke, L. (2001). Cortical Activations during the mental rotation of different visual objects. NeuroImage 13, 143–152. doi: 10.1006/nimg.2000.0677
Just, M. A., and Carpenter, P. A. (1976). Eye fixations and cognitive processes. Cogn. Psychol. 8, 441–480. doi: 10.1016/0010-0285(76)90015-3
Kaltner, S., Riecke, B. E., and Jansen, P. (2014). Embodied mental rotation: a special link between egocentric transformation and the bodily self. Front. Psychol. 5:505. doi: 10.3389/fpsyg.2014.00505
Ke, J., and Wang, L. (2021). Effects of time pressure and gender differences on mental rotation ability of novice drivers (in Chinese). Chin. J. Ergonom. 27, 12–21. doi: 10.13837/j.issn.1006-8309.2021.01.003
Kosslyn, S. M., DiGirolamo, G. J., Thompson, W. L., and Alpert, N. M. (1998). Mental rotation of objects versus hands: Neural mechanisms revealed by positron emission tomography. Psychophysiology 35, 151–161. doi: 10.1111/1469-8986.3520151
Kosslyn, S. M., Thompson, W. L., Wraga, M., and Alpert, N. M. (2001). Imagining rotation by endogenous versus exogenous forces: Distinct neural mechanisms. Neuroreport 12, 2519–2525. doi: 10.1097/00001756-200108080-00046
Lemke, M. R., Puhl, P., Koethe, N., and Winkler, T. (1999). Psychormotor retardation and anhedonia in depression. Acta Psychiat. Scandin. 99, 252–256. doi: 10.1111/j.1600-0447.1999.tb07221.x
Li, H., Liu, T., Woolley, J. D., and Zhang, P. (2019). Reality status judgments of real and fantastical events in children’s prefrontal cortex: An fNIRS study. Front. Human Neurosci. 13:444. doi: 10.3389/fnhum.2019.00444
Li, Y., and Shu, H. (2014). The brain mechanisms and functional hypothesis of default mode network and its clinical application (in Chinese). Adv. Psychol. Sci. 22, 234–249. doi: 10.3724/SP.J.1042.2014.00234
Luca, M., Luca, A., and Calandra, C. (2013). Psychomotor retardation and externally oriented thinking in major depression. Neuropsychiat. Dis. Treat. 9, 759–766. doi: 10.2147/NDT.S44650
Ma, Q., Hu, L., Li, J., Hu, Y., Xia, L., Chen, X., et al. (2016). Different effects of hypoxia on mental rotation of normal and mirrored letters: Evidence from the rotation-related negativity. PLoS One 11:e154479. doi: 10.1371/journal.pone.0154479
Ma, Y., Feng, H., Tang, Yi, Zhang, Y., and Li, C. (2009). Features of deactivation of depression in emotional cognitive tasks (in Chinese). Chin. J. Clin. Psychol. 17, 191–193. doi: 10.16128/J.cnki.1005-3611.2009.02.011
Mauri, M., Grazioli, S., Crippa, A., Bacchetta, A., Pozzoli, U., Bertella, S., et al. (2020). Hemodynamic and behavioral peculiarities in response to emotional stimuli in children with attention deficit hyperactivity disorder: An fNIRS study. J. Affect. Disord. 277, 671–680. doi: 10.1016/j.jad.2020.08.064
Mei, D. (2011). The influence from cognitive style and navigation type on maporientation performance (in Chinese). Hangzhou: Zhejiang Sci-Tech University.
Nishimura, Y., Takizawa, R., Muroi, M., Marumo, K., Kinou, M., and Kasai, K. (2011). Prefrontal cortex activity during response inhibition associated with excitement symptoms in schizophrenia. Brain Res. 1370, 194–203. doi: 10.1016/j.brainres.2010.11.003
Oshiyama, C., Sutoh, C., Miwa, H., Okabayashi, S., Hamada, H., Matsuzawa, D., et al. (2018). Gender-specific associations of depression and anxiety symptoms with mental rotation. J. Affect. Disord. 235, 277–284. doi: 10.1016/j.jad.2018.04.006
Ozel, S., Larue, J., and Molinaro, C. (2002). Relation between sport activity and mental rotation: comparison of three groups of subjects. Percep. Motor Skills 95, 1141–1154. doi: 10.2466/pms.2002.95.3f.1141
Paschke, K., Jordan, K., Wüstenberg, T., Baudewig, J., and Leo Müller, J. (2012). Mirrored or identical: Is the role of visual perception underestimated in the mental rotation process of 3D-objects: a combined fMRI-eye tracking-study. Neuropsychologia 50, 1844–1851. doi: 10.1016/j.neuropsychologia.2012.04.010
Paxton, J. L., Barch, D. M., Racine, C. A., and Braver, T. S. (2008). Cognitive control, goal maintenance, and prefrontal function in healthy aging. Cereb. Cortex 18, 1010–1028. doi: 10.1093/cercor/bhm135
Pinti, P., Scholkmann, F., Hamilton, A., Burgess, P., and Tachtsidis, I. (2019). Current status and issues regarding pre-processing of fNIRS neuroimaging data: An investigation of diverse signal filtering methods within a general linear model framework. Front. Human Neurosci. 12:505. doi: 10.3389/fnhum.2018.00505
Pizzagalli, D. A., and Roberts, A. C. (2021). Prefrontal cortex and depression. Neuropsychopharmacology 47, 225–246. doi: 10.1038/s41386-021-01101-7
Quan, C., Li, C., Xue, J., Yue, J., and Zhang, C. (2017). Mirror-normal difference in the late phase of mental rotation: An ERP study. PLoS One 12:e184963. doi: 10.1371/journal.pone.0184963
Rodrigo, A. H., Di Domenico, S. I., Ayaz, H., Gulrajani, S., Lam, J., and Ruocco, A. C. (2014). Differentiating functions of the lateral and medial prefrontal cortex in motor response inhibition. NeuroImage 85, 423–431. doi: 10.1016/j.neuroimage.2013.01.059
Rodríguez-Cano, E., Alonso-Lana, S., Sarró, S., Fernández-Corcuera, P., Goikolea, J. M., Vieta, E., et al. (2017). Differential failure to deactivate the default mode network in unipolar and bipolar depression. Bipol. Disord. 19, 386–395. doi: 10.1111/bdi.12517
Rogers, M. A., Bradshaw, J. L., Phillips, J. G., Chiu, E., Mileshkin, C., and Vaddadi, K. (2002). Mental rotation in unipolar major depression. J. Clin. Exp. Neuropsychol. 24, 101–106. doi: 10.1076/jcen.24.1.101.974
Schöning, S., Engelien, A., Kugel, H., Schäfer, S., Schiffbauer, H., Zwitserlood, P., et al. (2007). Functional anatomy of visuo-spatial working memory during mental rotation is influenced by sex, menstrual cycle, and sex steroid hormones. Neuropsychologia 45, 3203–3214. doi: 10.1016/j.neuropsychologia.2007.06.011
Shepard, R. N., and Metzler, J. (1971). Mental rotation of three-dimensional objects. Science 171, 701–703. doi: 10.1126/science.171.3972.701
Shmuel, A., Yacoub, E., Pfeuffer, J., Van de Moortele, P., Adriany, G., Hu, X., et al. (2002). Sustained negative BOLD, blood flow and oxygen consumption response and its coupling to the positive response in the human brain. Neuron 36, 1195–1210. doi: 10.1016/S0896-6273(02)01061-9
Singh, A. K., and Dan, I. (2006). Exploring the false discovery rate in multichannel NIRS. NeuroImage 33, 542–549. doi: 10.1016/j.neuroimage.2006.06.047
Toenders, Y. J., van Velzen, L. S., Heideman, I. Z., Harrison, B. J., Davey, C. G., and Schmaal, L. (2019). Neuroimaging predictors of onset and course of depression in childhood and adolescence: A systematic review of longitudinal studies. Dev. Cogn. Neurosci. 39:100700. doi: 10.1016/j.dcn.2019.100700
Vingerhoets, G., de Lange, F. P., Vandemaele, P., Deblaere, K., and Achten, E. (2002). Motor imagery in mental rotation: An fMRI study. NeuroImage 17, 1623–1633. doi: 10.1006/nimg.2002.1290
Wang, L., and Sang, B. (2014). Embodied cognition of concepts (in Chinese). J. Nantong Univ. 30, 100–106.
Wang, Z., Yuan, C., Huang, J., Li, Z., Chen, J., Zhang, H., et al. (2011). Reliability and validity of the Chinese version of Beck Depression Inventory-? among depression patients. Chin. Mental Health J. 25, 476–480. doi: 10.3969/j.issn.1000-6729.2011.06.014
Wriessnegger, S. C., Bauernfeind, G., Schweitzer, K., Kober, S., Neuper, C., and Müller-Putz, G. (2012). The interplay of prefrontal and sensorimotor cortices during inhibitory control of learned motor behavior. Front. Neuroengineer. 5:17. doi: 10.3389/fneng.2012.00017
Wu, D., Yang, J., Xie, S., Luo, J., Chang, C., and Li, H. (2020). An fNIRS examination of the neural correlates of mental rotation in preschoolers. Human Behav. Brain 1, 37–42. doi: 10.37716/HBAB.2020010201
Yan, C., Chen, X., Li, L., Castellanos, F. X., Bai, T., Bo, Q., et al. (2019). Reduced default mode network functional connectivity in patients with recurrent major depressive disorder. Proc. Nat. Acad. Sci. 116, 9078–9083. doi: 10.1073/pnas.1900390116
Yan, J., Sun, J., Guo, X., Jin, Z., Li, Y., Li, Z., et al. (2013). Motor imagery cognitive network after left ischemic stroke: Study of the patients during mental rotation task. PLoS One 8:77325. doi: 10.1371/journal.pone.0077325
Yan, W., Zhang, M., and Liu, Y. (2021). Regulatory effect of drawing on negative emotion: a functional near-infrared spectroscopy study. Arts Psychother. 74:101780. doi: 10.1016/j.aip.2021.101780
Yang, W., Liu, S., Zhou, T., Peng, F., Liu, X., Li, L., et al. (2014). Reliability and validity of chinese version of the beck depression inventory-? in Chinese adolescents (in Chinese). Chin. J. Clin. Psychol. 22, 240–245. doi: 10.16128/j.cnki.1005-3611.2014.02.018
Ye, J. C., Tak, S., Jang, K. E., Jung, J., and Jang, J. (2009). NIRS-SPM: Statistical parametric mapping for near-infrared spectroscopy. NeuroImage 44, 428–447. doi: 10.1016/j.neuroimage.2008.08.036
Zhang, P., Zhang, Q., Li, S., Song, L., Yang, Y., and Bai, X. (2020). The effect of anticipatory punishment on cognitive control: Behavioral and fNIRS evidence (in Chinese). J. Psychol. Sci. 43, 534–541. doi: 10.16719/j.cnki.1671-6981.20200304
Keywords: mental rotation, depression, fNIRS, psychomotor retardation, subject mental rotation, object mental rotation, mental rotation with mirrored stimuli
Citation: Wang L, Ke J and Zhang H (2022) A Functional Near-Infrared Spectroscopy Examination of the Neural Correlates of Mental Rotation for Individuals With Different Depressive Tendencies. Front. Hum. Neurosci. 16:760738. doi: 10.3389/fnhum.2022.760738
Received: 26 August 2021; Accepted: 17 January 2022;
Published: 07 February 2022.
Edited by:
Vilfredo De Pascalis, Sapienza University of Rome, ItalyCopyright © 2022 Wang, Ke and Zhang. This is an open-access article distributed under the terms of the Creative Commons Attribution License (CC BY). The use, distribution or reproduction in other forums is permitted, provided the original author(s) and the copyright owner(s) are credited and that the original publication in this journal is cited, in accordance with accepted academic practice. No use, distribution or reproduction is permitted which does not comply with these terms.
*Correspondence: Haiyan Zhang, hldhaiyan@163.com